Jump to a key chapter
Understanding the Concept of Viral Genome
Let's dive right into the intriguing world of microbiology where the viral genome is a star player. The viral genome is a critical element in the life cycle of a virus. Acting as the carrier for the virus's genetic information, it provides you with a unique perspective on how viruses reproduce and function.Viral Genome: A Definition
To appreciate the journey of understanding a viral genome, it's essential to start with its definition.A viral genome refers to the complete set of genetic material within a virus. This genetic material can be either DNA (Deoxyribonucleic Acid) or RNA (Ribonucleic Acid), and its structure can be either single-stranded or double-stranded.
While cells predominantly have double-stranded DNA as their genetic material, viruses showcase much more variety. From single-stranded RNA to double-stranded RNA, and from single-stranded DNA to double-stranded DNA, viruses exhibit remarkable genetic diversity.
Key Functions of a Viral Genome
You might be wondering what role the viral genome plays within a virus' lifecycle. There are a few key functions this small yet potent piece of material serves.- Coding Proteins: A crucial function of the viral genome is to code for viral proteins. These proteins aid in the creation of new virus particles.
- Self-Replication: Some types of viral genome can replicate independently by using their own replications enzymes.
- Invading Host Cells: The viral genome contains the information needed for a virus to invade host cells, reproduce, and continue its life cycle.
The Structure of Viral Genome: An Overview
As an example of a viral genome, consider the Influenza A virus. It has a segmented negative-sense single-stranded RNA genome. This means that its genome is separated into several segments, each encoding different proteins.
Genome Type | Example Virus |
Double-stranded DNA | Herpesviruses |
Single-stranded DNA | Parvoviruses |
Double-stranded RNA | Reoviruses |
Single-stranded RNA | Influenza Virus |
7 Types of Viral Genomes
Ready to uncover more about the viral world? A fascinating aspect of viral biology lies in the assortment of their genome types. Unlike cellular life forms, which predominantly harness double-stranded DNA (dsDNA), viruses exhibit an impressive range of genome types. These seven types are classified primarily based on the kind of nucleic acid (DNA or RNA), its structure (single or double-stranded), and the sense of the genome (positive or negative sense).The Classification and Implications of Different Viral Genomes
As we delve into the types of viral genomes, it is essential to classify them efficiently. Embarking on this journey, we need to familiarise ourselves with a few key terms. Here they are:- DNA: Deoxyribonucleic Acid
- RNA: Ribonucleic Acid
- dsDNA: Double-Stranded DNA
- ssDNA: Single-Stranded DNA
- dsRNA: Double-Stranded RNA
- ssRNA: Single-Stranded RNA
- Positive-sense: Single-stranded RNA viruses with genome akin to mRNA
- Negative-sense: Single-stranded RNA viruses with genomes complementary to mRNA
An Insight into These Varied Types of Genomes
Unravelling the intricate implications of the seven viral genome types, we are prompted to delve into a deeper understanding of each one. Let's look at some representative viruses for each type of genome:Genome Type | Virus |
DsDNA | Herpes simplex virus |
SsDNA | Parvovirus B19 |
dsDNA-RT | Hepatitis B virus |
SsRNA-RT | HIV |
dsRNA | Rotavirus |
Positive-sense SsRNA | SARS-CoV-2 |
Negative-sense SsRNA | Influenza virus |
Unravelling the Viral Genome Replication Process
The world of viruses is a fascinating one, rich in diversity and teeming with intriguing processes. Among these, one aspect that captures attention is the replication processes of viral genomes, also known as the multiplication cycle of viruses. There are a number of stages involved in this process, which we will delve into in the following sections.The Key Stages in Viral Genome Replication
Let's now explore the four key steps within the viral genome replication cycle:- Entry
- Biosynthesis
- Maturation
- Release
Factors Influencing the Viral Genome Replication
Multiple factors can influence the efficiency and the mode of this viral genome replication process. Let's delve into some of the most significant ones:- Virus Type: The type of virus is a crucial determining factor. As we know, viruses can be classified into seven groups based on their genome type and replication strategy, each with its unique characteristics and methods of genome replication. For instance, the replication process of a DNA virus is vastly different from that of an RNA virus, not to mention the differences among DNA viruses themselves.
- Host Cell Type: Another essential factor is the type of host cell. Different types of cells possess different receptors on their surface, which can influence the entry of the virus. They can also vary in terms of their machinery for replication and protein synthesis, which can subsequently impact the stages of biosynthesis and maturation. The condition of the host cell, such as whether it is in a state of growth or division, can also influence viral genome replication.
- Environmental Conditions: The conditions in which the virus and the host cell exist can also play a role in the replication process. This includes factors such as temperature, pH, and the presence of certain chemicals. These conditions can affect not only the viability of the virus but also the activity of the host cell and its interaction with the virus.
Decoding the Process and Importance of Viral Genome Sequencing
In unravelling the mysteries of microbiology, one powerful tool we harness is viral genome sequencing. But what precisely does viral genome sequencing entail, and why is it so instrumental in understanding the microscopic world of viruses?What is Viral Genome Sequencing?
Viral genome sequencing uncovers the exact order of nucleotides within a viral genome. For context, a virus's genome, composed of nucleic acids (either DNA or RNA), serves as a code that instructs how to assemble and operate the virus. These nucleic acids are strings of molecules, termed nucleotides, linked together like beads on a string. Within these nucleotides, four types exist: Adenine (A), Cytosine (C), Guanine (G), and Thymine (T) or Uracil (U). Adenine always pairs with Thymine (in DNA) or Uracil (in RNA), and Cytosine always associates with Guanine, forming the basis of this genetic code. Sequencing the viral genome involves identifying the precise sequence of nucleotides. Capturing this sequence avails a complete roadmap of the virus's genetic composition, which can then be analysed for specific attributes and compared to other viral sequences. This process is made possible by sophisticated techniques such as Sanger sequencing, or more commonly, Next-Generation Sequencing (NGS).For instance, consider a simple linear ssRNA virus with a genome length of 10 nucleotides. A possible sequenced genome could look like this: 'AUGGCUAACU'. Each nucleotide in this viral genome holds pivotal information, playing roles in virus replication, transcription, and translation.
The Role it Plays in Understanding Microbiology
Implementing viral genome sequencing in microbiology is akin to possessing a guidebook to navigate the intricate labyrinth of viral genetics, as it plays a central role in various capacities. Let's break down some of its most crucial functions:- Virus Identification A significant stride genome sequencing has enabled in microbiology is precise viral identification. Through genome sequencing, we can distinguish viruses at the strain level, enabling us to differentiate between closely related virus strains and types. This technique is crucial for diagnosing infections, monitoring virus evolution, and tracking outbreaks.
- Understanding Virus Evolution and Phylogeny Through genome sequencing, we get a glimpse of the virus's evolutionary history. By comparing the genome sequences of different viruses, we can deduce their phylogenetic relationships, understand how they have evolved over time, and predict their future evolution.
- Vaccine and Antiviral Development Sequence information is pivotal to the development of vaccines and antiviral drugs. For instance, sequencing the genome of the influenza virus each year helps researchers predict which strains will be predominant in the next flu season, aiding in the development of that year's flu vaccine.
- Informed Public Health Decisions Genome sequencing has been instrumental in responding to viral outbreaks. For instance, during the COVID-19 pandemic, sequencing SARS-CoV-2 genomes has helped track the virus's spread and evolution, monitor the emergence of new variants, and inform public health decision-making.
Consider the case of the H1N1 Influenza A virus that caused the 2009 pandemic. Genome sequencing was crucial in understanding the virus's origins and how its genetic makeup allowed it to jump from animals to humans. It also enabled scientists to monitor its global spread and facilitated the swift development of a vaccine.
Largest and Defective Viral Genomes: A Comparative Study
Understanding the Largest Viral Genomes
Delving into the realm of microbiology often uncovers striking contrasts, one of them being the significant differences in the size of viral genomes. While they all essentially perform the same function of encoding the virus's instructions and identity, the range in their sizes is considerable, with the largest viral genomes reaching impressive lengths. So, what precisely constitutes the largest viral genomes? The answer lays in a unique subset of viruses known as the Mimiviridae family. This family predominantly includes giant viruses such as the Mimivirus and the Pandoravirus, which boast some of the largest viral genomes known to science. Scientists have found that genomes of these giant viruses can reach up to 1.5 million base pairs (Mbp), dwarfing many other viral genomes. To put this into perspective, the genome of the SARS-CoV-2 virus responsible for the COVID-19 pandemic is approximately 0.03 Mbp.Mimiviridae is a family of viruses known for their large size and complex genomes. First discovered in 1992, they have since intrigued scientists by challenging the traditional definitions and assumptions about viruses.
A Closer Look at Defective Viral Genomes
Now, let's explore the other end of the spectrum, where we encounter what are known as 'defective' viral genomes. These genomes are characterised by deletions, mutations, or other alterations that render them incapable of producing fully functional virions in the absence of a helper virus. As such, they represent a somewhat paradoxical aspect of virology, being "defective" yet often playing significant roles in virus-host interactions and viral pathogenesis. An intriguing class of defective viral genomes (DVGs) is deficient interfering particles (DIPs). DIPs are produced when the viral replication machinery makes errors, resulting in the production of genomes that lack one or more essential genes. Crucially, DIPs can still replicate if a functional virus is present within the same cell, hence they 'interfere' with the replication of the standard virus by competing for resources. Defective viral genomes differ in various types of viruses. In RNA viruses, for instance, DVGs commonly involve considerable deletions, often removing significant proportions of the genome. In contrast, DVGs in DNA viruses frequently involve rearrangements, such as inversions, duplications, or translocations.An example of a virus known for generating DIPs is the Influenza A Virus (IAV). Here, DIPs have been observed to carry deletions in their genomic segments. These DIPs can significantly interfere with the replication of full-length influenza viruses, thereby influencing viral pathogenesis and the host immune response.
The Consequences and Causes of Defective Viral Genomes
Understanding defective viral genomes is crucial, not just because they represent a significant proportion of viral particles in an infected host but also because of their potential implications on the infection outcome and host-virus interactions. DVGs and DIPs can significantly affect the pathogenicity and transmission of viruses. For instance, the presence of DIPs can interfere with the replication of infectious viruses, thereby modulating the severity of the infection. They can also play a role in modulating the host's immune response. What causes defective genomes to arise? One primary factor is the error-prone nature of viral replication, particularly in RNA viruses. As RNA replication lacks the proofreading mechanisms found in DNA replication, errors such as deletions and mutations occur more frequently. Other factors like host immunity and viral population dynamics can influence the production and selection of defective genomes.Delving into the topic, it is found that studies on Hepatitis C Virus (HCV) have revealed a positive correlation between the presence of defective viral genomes and a host's response to antiviral therapy, hinting at possible practical applications of studying DVGs. There's been growing interest in harnessing DIPs or DVGs as potential antiviral therapeutics, leveraging their ability to interfere with viral replication.
Viral Genome - Key takeaways
Key Takeaways
- Viral genomes can be classified into seven types: dsDNA, ssDNA, dsDNA-RT, ssRNA-RT, dsRNA, positive-sense ssRNA, and negative-sense ssRNA, with differential replication strategies linked to the viral lifecycle.
- DNA viruses (such as dsDNA and ssDNA) usually replicate in the host's nucleus, whereas RNA viruses (like dsRNA and ssRNA) may replicate in viral cores within the host cytoplasm or operate as mRNA for instant protein translation.
- The viral genome replication process comprises four key steps: Entry, Biosynthesis, Maturation, and Release, each having significance in how a virus multiplies and spreads within host cells. The process may differ among viruses based on their genome type and replication strategy.
- Viral genome sequencing is a technique that identifies the exact sequence of nucleotides (A, C, G, and T or U) within a viral genome. It provides critical insights into the virus's genetic composition, helping in virus identification, understanding evolution, vaccine development, and informed public health decisions.
- Largest viral genomes are found in the Mimiviridae family, with genomes reaching up to 1.5 million base pairs (Mbp). On the other hand, defective viral genomes (not discussed in the input text) are incomplete or mutated versions of full-length genomes which may influence virus transmission and pathogenesis.
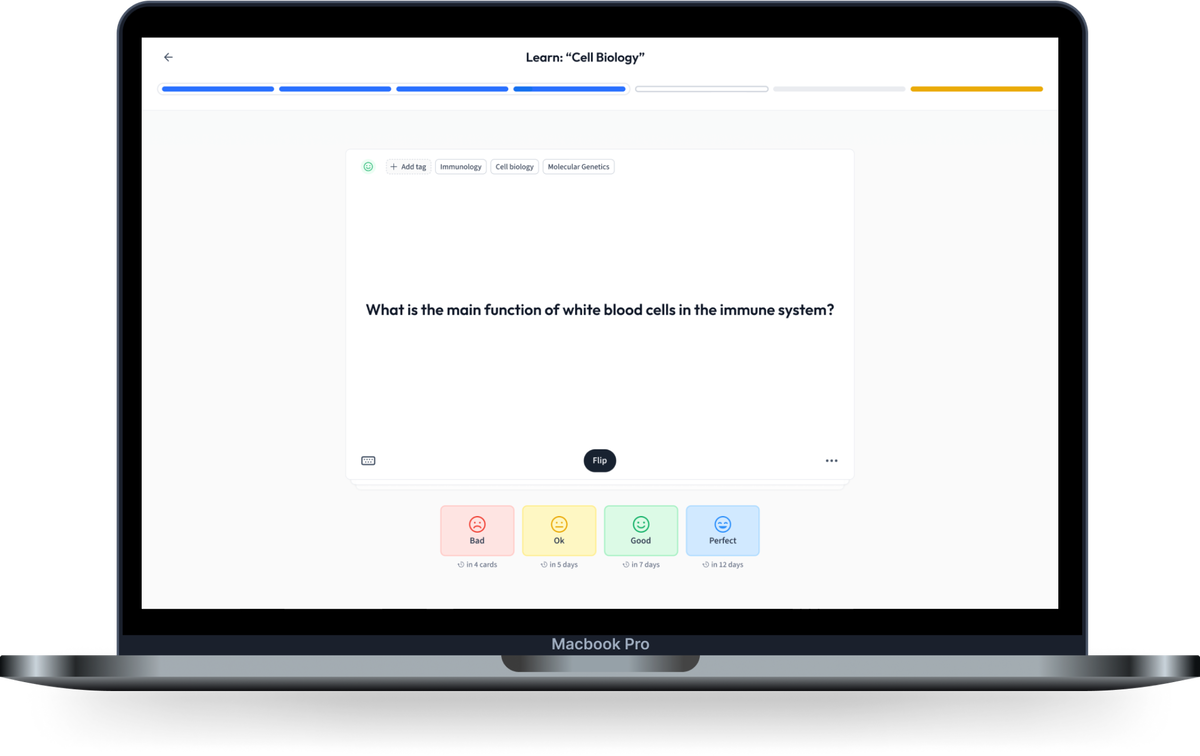
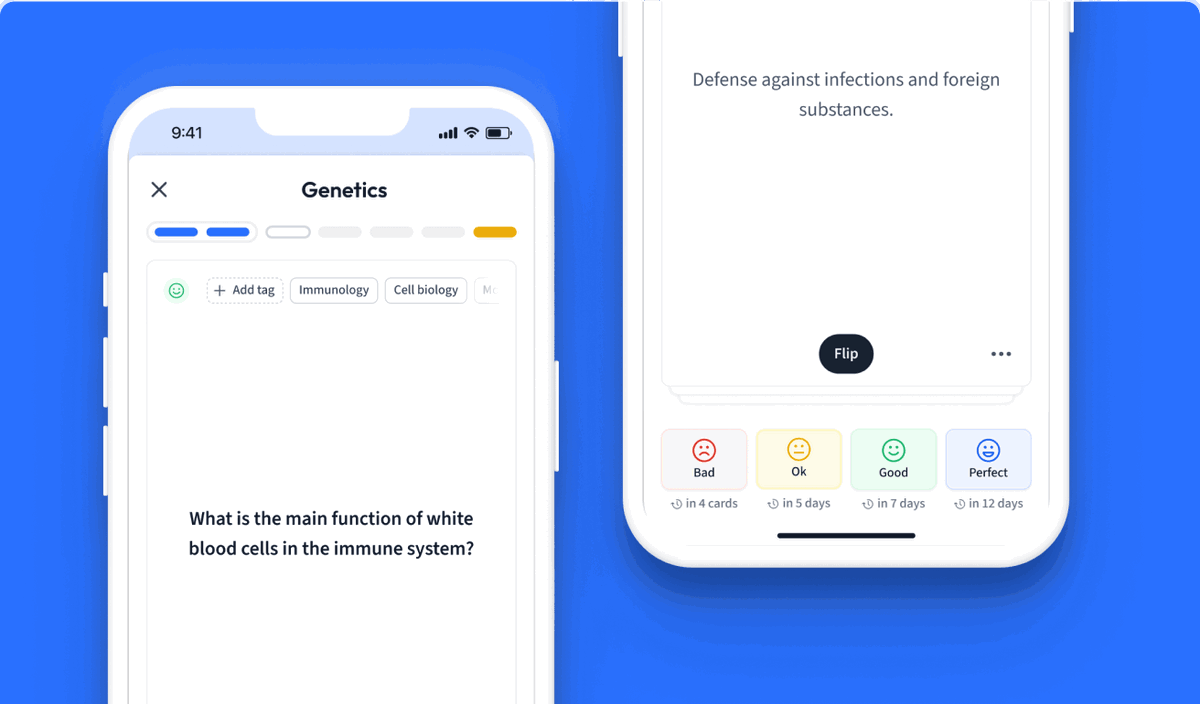
Learn with 15 Viral Genome flashcards in the free StudySmarter app
We have 14,000 flashcards about Dynamic Landscapes.
Already have an account? Log in
Frequently Asked Questions about Viral Genome
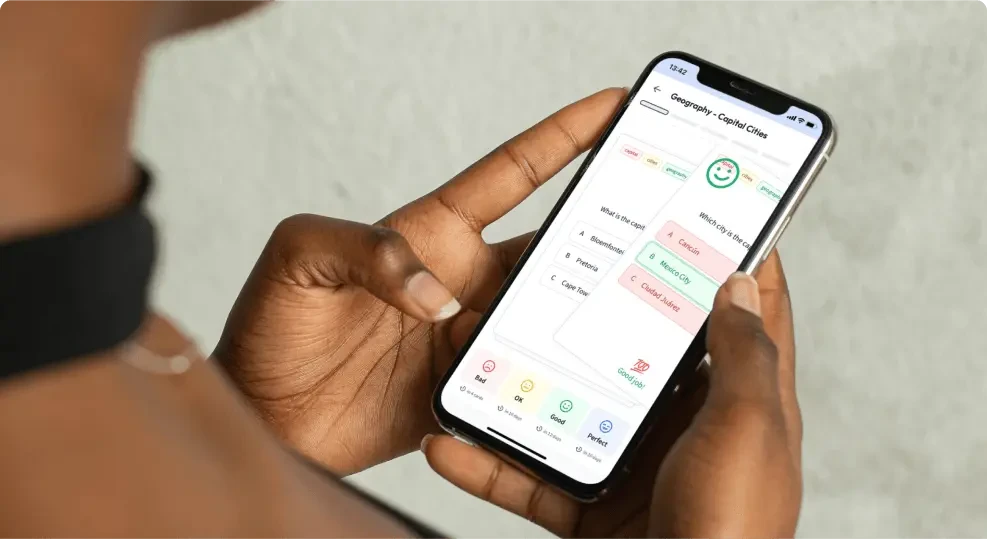
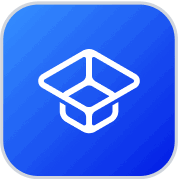
About StudySmarter
StudySmarter is a globally recognized educational technology company, offering a holistic learning platform designed for students of all ages and educational levels. Our platform provides learning support for a wide range of subjects, including STEM, Social Sciences, and Languages and also helps students to successfully master various tests and exams worldwide, such as GCSE, A Level, SAT, ACT, Abitur, and more. We offer an extensive library of learning materials, including interactive flashcards, comprehensive textbook solutions, and detailed explanations. The cutting-edge technology and tools we provide help students create their own learning materials. StudySmarter’s content is not only expert-verified but also regularly updated to ensure accuracy and relevance.
Learn more