Understanding the BAC: Introduction to Bacterial Artificial Chromosomes
Take a moment to dive into the fascinating world of microbiology. Today, you will learn about BAC, or Bacterial Artificial Chromosomes, which are crucial tools used in genetics and genomics research.BAC, short for Bacterial Artificial Chromosomes, are DNA constructs, created by scientists, used to clone DNA sequences for genetic study.
Breaking Down the BAC Meaning
To fully understand the term BAC, it's helpful to break it down:- Bacterial: This refers to the bacterial origin of the DNA used to create the artificial chromosome.
- Artificial: These are man-made constructs, created in a lab setting.
- Chromosome: This is the DNA molecule which carries genetic information.
The process by which bacteria replicate DNA is called bacterial conjugation, which is an essential process in bacterial genetics. It allows scientists to produce large quantities of a specific DNA sequence in a short time.
Origin and Structure of BAC: Decoding the Bacterial Artificial Chromosome
Originally developed in the 1990s, BACs were created to aid in the process of sequencing entire genomes, including the Human Genome Project.Year of Development | Main Purpose |
1990s | Sequencing entire genomes |
- A selectable marker gene which allows for the identification of successful transformants.
- An origin of replication which triggers DNA replication within the bacterial cell.
- Multiple cloning sites where the DNA of interest can be inserted.
- A restriction site which allows the BAC to be cut open.
For instance, if you wanted to study a gene that you suspect might be involved in a certain disease, you would insert that gene's DNA into the BAC's multiple cloning site. Upon introducing the BAC into a bacterial cell, the cell's replication machinery would start mass-producing this gene, providing you with an ample supply for analysis and experimentation.
- Large DNA fragments can be inserted, making them ideal for studying large genes or genomic regions.
- Their high stability ensures that the cloned DNA will remain intact without unwanted rearrangements.
- They can be easily purified from the bacterial cells, facilitating downstream genetic analyses.
Functions and Applications of BAC in Microbiology
BACs are not just mere tools, they play some very significant roles in the field of microbiology. Their ability to hold large fragments of DNA and maintain them stably makes them valuable resources in various biological studies.The BAC Cloning Process
The BAC cloning process can be quite detailed but understanding it can help appreciate the exact functioning of BACs. The process starts by using restriction enzymes to cut the DNA fragment of interest from the source genome. Restriction enzymes function like molecular scissors, able to recognise and cleave DNA at specific sequences, known as restriction sites. Simultaneously, the BAC is also cut open at its corresponding restriction site that matches the one on the DNA fragment to be cloned. Both the DNA fragment and BAC are given appropriate 'ends' using these enzymes so they can fit together like pieces of a puzzle. This is followed by a process known as ligation, where the DNA fragment and BAC are attached together by the enzyme DNA ligase to form a recombinant DNA molecule, that is, a BAC with the target DNA fragment. After this, the recombinant BAC must be introduced into bacterial cells, a process known as transformation. Once inside the bacterial cell, the recombinant BAC utilises the cell's machinery for DNA replication to multiply. Each time the bacterial cell replicates, the BAC clone is duplicated too, leading to the production of multiple copies of the cloned DNA fragment. Now researchers can grow huge amounts of these bacteria and harvest the cloned DNA for further analyses. In addition, the BAC also carries genes that provide resistance to certain antibiotics. So, growing the transformed bacteria on a medium containing those antibiotics allows the selection of only those cells that contain the BAC. It's important to calculate the probability of finding the desired clone in a BAC library. This probability \[ P \] can be calculated using the formula, \[ P = 1 - e^{-n} \] where \( e \) is the base of the natural logarithm, and \( n \) is the number of recombinant clones. This equation shows the probability of finding your gene of interest in your BAC library.What are Bacterial Artificial Chromosomes Used for in Microbiology?
BACs have a multitude of applications in microbiology, holding a central position in genetic and genomic research. Let's explore some of the key uses. Firstly, BACs have been a cornerstone in genome sequencing projects such as the Human Genome Project. They have helped in determining the sequences of large genomes piece by piece. After the entire genome of the organism is broken down and inserted into separate BACs to create a BAC library, each BAC is then sequenced separately, and finally, the sequences are assembled to derive the complete genome sequence. Secondly, BACs find use in gene discovery and functional studies. For instance, they allow researchers to study the influence of surrounding DNA sequences on gene expression. Moreover, genetically modified organisms (GMOs) are often created using BACs, which allow for the insertion of large pieces of foreign DNA into a host organism. The study of specific complex traits and gene families is another application. Given that BACs can carry large stretches of DNA, they can encompass an entire gene family or large genomic region responsible for a complex trait. This can provide invaluable insights into the genetic basis of complicated biological phenomena. One very popular use of BACs is in Creating Transgenic Mice. BAC transgenic mice can be generated by injecting the relevant BAC clone into the pronucleus of a fertilized mouse oocyte. Finally, BACs are also used in comparative genomics studies to highlight the genetic differences between species, which can in turn shed light on their evolutionary relationships. The importance of BACs in microbiology therefore, is manifold. They have been instrumental, not just in expanding our knowledge of genetics, but also in creating new ways of applying this knowledge for the development of better diagnostics, therapies and other biomedical applications.Exploring the BAC: Advantages and Disadvantages
In any discussion involving the use of bacterial artificial chromosomes (BAC), it is essential to consider both the advantages and disadvantages. Just as with any tool in science, BACs have their strengths and limitations, depending on the context of the experiment.Bacterial Artificial Chromosome Advantages in Biology Experiments
There are several reasons why BACs are often the preferred choice for scientists in cloning large segments of DNA. First and foremost, BACs can accommodate larger fragments of DNA than other vectors. This ability makes it possible for scientists to clone entire genes, even ones with large introns, or large operons which are groups of genes that function together. Secondly, BACs are stable and tend to maintain the inserted DNA sequence without any major rearrangements or deletions. This is especially useful for scientists who need to be certain the DNA they are studying hasn't been altered during the cloning process. Thirdly, BACs replicate independently of the bacterial chromosome in host cells, resulting in a single copy of a BAC in each cell after replication. Such low copy numbers significantly reduce the chance of recombination events, hence maintaining the integrity of the cloned DNA. Fourthly, BACs allows for the propagation of very large DNA molecules. This is a capability that other types of vectors, like plasmids and cosmids, lack. Fifthly, BACs have a large cloning capacity, typically allowing the cloning of DNA fragments that are between 100 and 300 kilobases long. This makes BACs an attractive choice for creating genomic libraries for genome sequencing and mapping. These advantages boil down to three key points:- Large insert capacity
- High-level stability
- Low copy number
The Downsides of Using Bacterial Artificial Chromosomes
In spite of their various benefits, BACs do come with some setbacks which can limit their utility in certain experimental contexts. One significant limitation is that BACs require a more elaborate process for the insertion and propagation of DNA fragments compared to simpler vectors like plasmids. This requirement can slow down the pace of research and increase the complexity of experimental procedures. Secondly, BACs are not suitable for expressing cloned genes in host cells due to their low copy number. Low copy number means that only a few copies of the DNA are present in each cell, which limits the quantity of protein that can be produced from a gene within the BAC. Thirdly, selecting the right clone from a library can be more challenging with BACs because of their larger insert capacity. More DNA means more genes, and thus, more potential for selecting a clone of interest. Fourthly, BACs suffer from a relatively lower efficacy during transformation (the process of introducing the BAC into bacterial cells) which makes the process more cumbersome. Lastly, the constructs are large and difficult to purify from bacterial cells. This can make downstream analyses more complicated or require more sophisticated laboratory methods. In summary, the disadvantages of BACs include:- More complex manipulation and propagation procedures
- Low gene expression in host cells
- Challenges in selecting the right clone from a library
- Lower transformation efficiency
- Difficulty in purifying the constructs
Detailed Look at the Bacterial Artificial Chromosome Structure
Before delving into the complexities and specifics of genetic cloning, it's worth first understanding what a bacterial artificial chromosome (BAC) is made up of. A typical BAC comprises several essential sections that aid in its functioning.Key Components of Bacterial Artificial Chromosome Structure
Bacterial artificial chromosomes are a type of plasmid - circular pieces of DNA that are separate from the bacterial chromosome. They are engineered for the cloning of large DNA fragments in bacterial cells. The structure of a BAC includes several key features that not only facilitate the integration of the DNA fragment, but also help in maintaining the clone once it's inside the bacterial cell.Origin of Replication (oriV): This is a sequence of DNA where replication of the plasmid commences. Plasmids with a certain origin of replication, derived from the F-factor plasmid of E. coli, lead to the BAC being present at low copy numbers in the bacterial cell, which is advantageous for maintaining larger DNA fragments.
Antibiotic Resistance Gene: This gene helps in the selection of successfully transformed bacterial cells. Cells that have incorporated the BAC can survive in the presence of a specific antibiotic due to the expression of this gene, while cells that haven't incorporated the BAC do not survive.
Cloning Site: Also known as the multiple cloning site (MCS), this region contains several unique restriction sites where the DNA fragment of interest can be inserted.
LacZ Gene: The lacZ gene plays a role in blue/white screening, which is a technique used to distinguish cells that have incorporated the BAC with an insert from those that have incorporated the BAC without an insert.
In a typical scenario, a scientist would extract DNA from the organism of interest and insert it into the cloning site of the BAC while in a solution outside of the bacterial cell. The antibiotic-resistant gene in the BAC is only active when the BAC is inside a bacterial cell, allowing only those cells that have incorporated the BAC to survive in the presence of that particular antibiotic. Meanwhile, the LacZ gene facilitates successful cloning by showing visual representation of the incorporated plasmid through blue/white screening.
Bacterial Artificial Chromosome Structure vs Other Vector Structures
Comparing with other popular vectors for DNA cloning - plasmids, cosmids, and YACs (Yeast Artificial Chromosomes), BACs come with a significant advantage: their large cloning capacity. While plasmids and cosmids can only carry a DNA fragment size of ~10kb and 40 kb respectively, BACs can carry DNA fragments that are up to 200 kb long, showcasing their capability to maintain large fragments of DNA. Additionally, BACs and YACs share a structural similarity - both of them have a low copy number within the cells. This, along with the above-mentioned larger fragment capacity, results in increased clone stability, which proves to be a big advantage in genomics studies, reducing the chances of DNA rearrangement or deletion. However, BACs are not without their limitations. The process of DNA insertion into BACs is complex due to the large DNA fragment sizes. Also, unlike plasmids, which usually carry a high copy number, BACs are single copy, limiting the quantity of DNA or proteins that can be produced. In conclusion, the utility of a BAC, plasmid, cosmid, or YAC is mainly dependent upon the specific needs and objectives of the experiment. Scientists would need to weigh the advantages and disadvantages of each vector system to determine which is most suitable for their particular study. While BACs offer a high capacity and stability for large DNA fragments, researchers must also consider their limitations. For smaller DNA fragments or when high quantities of DNA are needed, other vector systems might be more appropriate.BAC as Vectors: Understanding Bacterial Artificial Chromosomes
Bacterial artificial chromosomes (BAC) are engineered plasmids designed specifically to clone large fragments of DNA in bacterial cells. They have proven to be a powerful tool in molecular biology, enabling scientists to manipulate larger and more complex fragments of DNA than was previously possible, aiding in numerous advancements in genetic and genomic research.Role of Bacterial Artificial Chromosomes in Gene Therapy and Cloning
Gene therapy is a novel field of medicine that aims to treat or prevent diseases by introducing, deleting, or altering genes within a person's cells. BAC vectors play a significant role in facilitating this process due to their large capacity for DNA insertion. One significant application of BACs in gene therapy is their use in delivering large multi-gene loci to treat genetic diseases. These large genetic payloads need to be delivered to cells with precision and efficiency, and BACs, with their high stability and lower tendency for rearrangement, fulfill this purpose effectively. Another key use of BACs in gene therapy is in DNA vaccination. DNS vaccines are at the frontier of preventative medicine, representing a simple and low-cost approach to stimulate a potent, long-lasting immune response. BACs, being able to hold large DNA fragments, provides the means for DNA vaccines to carry multiple antigen genes. In the realm of genomic cloning, BACs have become almost indispensable. Owing to their ability to maintain large fragments of DNA with high fidelity, they have been extensively used in creating genomic libraries for gene discovery and complete genome mapping, including the Human Genome Project. When it comes to functional genomic studies, BACs step in as platforms for generating transgenic animals. They allow for a section of DNA, large enough to contain a complete gene along with its regulatory elements, to be integrated into the genome of a laboratory animal model. This approach has contributed significantly to unraveling the mysteries of gene function and regulation.It's important to note that while BACs have many strengths that make them suitable for gene therapy and cloning, it's crucial to bear in mind their limitations. The efficiency of BAC-mediated transformation may sometimes be lower than desired, their manipulation requires specific technical expertise, and their large size creates a barrier in the delivery to some types of cells. Despite these challenges, BACs have been a game-changer in many aspects of gene therapy and cloning, and researchers continue to innovate to overcome these limitations and expand their potential applications.
Potential Applications and Future Directions for Bacterial Artificial Chromosomes
In the world of genomics held by an ever-tightening grasp, BACs hold promise to steer countless scientific advancements. Human genetic disorder research is one such field benefiting largely from BAC-based studies. The production of transgenic mice carrying human genes within BACs is a robust method for studying gene function in human genetic disorders. As our understanding of human genetic diseases advances, the availability of BAC-based tools to study the underlying genetics will only increase in significance. Research in pharmaceuticals is also bound to reap amazing benefits from BAC vectors. Whether it is the creation of DNA vaccines or the large-scale production of proteins for therapeutic uses, BACs offer an effective and safe method for manipulating and delivering DNA. Excitement also surrounds the potential application of BACs in agriculture. By genetically manipulating crops with BAC vectors, we might improve crop drought tolerance, pest resistance, and increase overall crop yield. In the field of genomics, the use of BACs in the construction of physical maps of genomes continues to contribute to emerging genome sequencing efforts. Furthermore, the use of BACs to clone and express genes in context continues to be a valuable approach for functional genomics research. The future possibilities for BACs are indeed extremely broad – from advancements in gene therapy, therapeutics' production, genome mapping, functional genomics, to even biotechnology and agriculture. As technology advances and our ability to manipulate and fine-tune these vectors improves, BACs are poised to continue playing a significant role in shaping the future of genetic research.BAC - Key takeaways
- Bacterial Artificial Chromosomes (BAC): Engineered plasmids designed to clone large fragments of DNA in bacterial cells, making them crucial for the analysis of larger and complex DNA sequences.
- BAC Cloning Process: Involves cutting the DNA fragment of interest from the source genome using restriction enzymes, attaching it to the BAC through a process called ligation, and introducing established recombinant DNA molecule into bacterial cells through transformation. BACs replicate in the bacterial cells, creating multiple copies of the cloned DNA for further analyses.
- Applications of BACs in Microbiology: BACs play a major role in genome sequencing projects, gene discovery, functional studies, study of complex traits, gene families, creation of transgenic mice, and in comparative genomics studies. BACs can hold a large amount of DNA and maintain this in a stable manner.
- Advantages and Disadvantages of BACs: While the advantages include large DNA fragment accommodation, high stability, and low chance of recombination events, the downsides include more complex manipulation and propagation procedures, challenges in selecting the right clone from a library, low gene expression in host cells, and lower transformation efficiency.
- Structure of BAC: A typical BAC includes an Origin of Replication (oriV), Antibiotic Resistance Gene, Cloning Site (multiple cloning site - MCS), LacZ Gene. These elements facilitate the integration of the DNA fragment and help in maintaining the clone inside the bacterial cell.
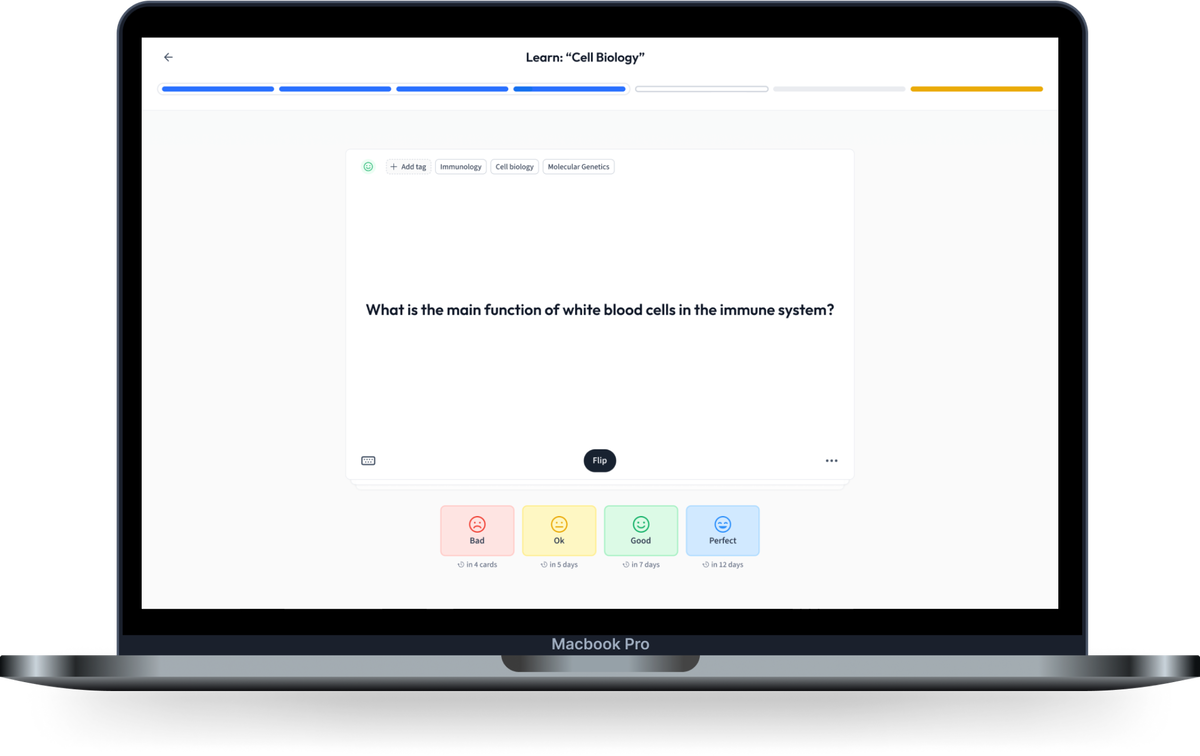
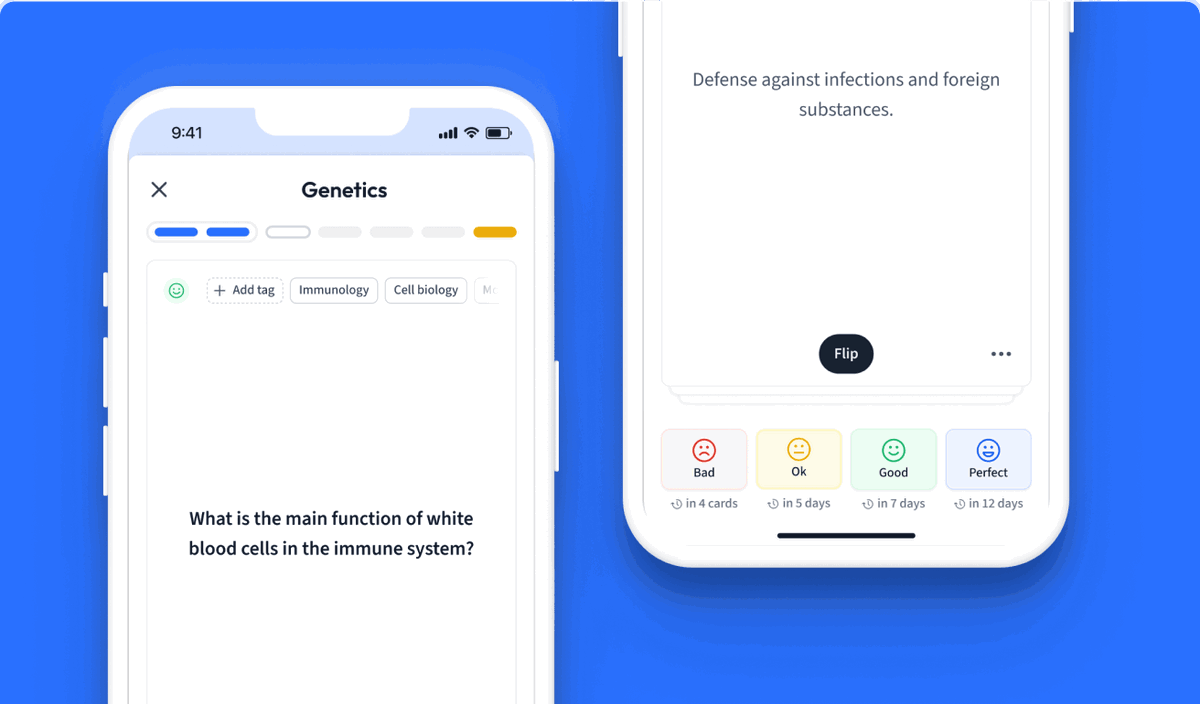
Learn with 15 BAC flashcards in the free StudySmarter app
We have 14,000 flashcards about Dynamic Landscapes.
Already have an account? Log in
Frequently Asked Questions about BAC
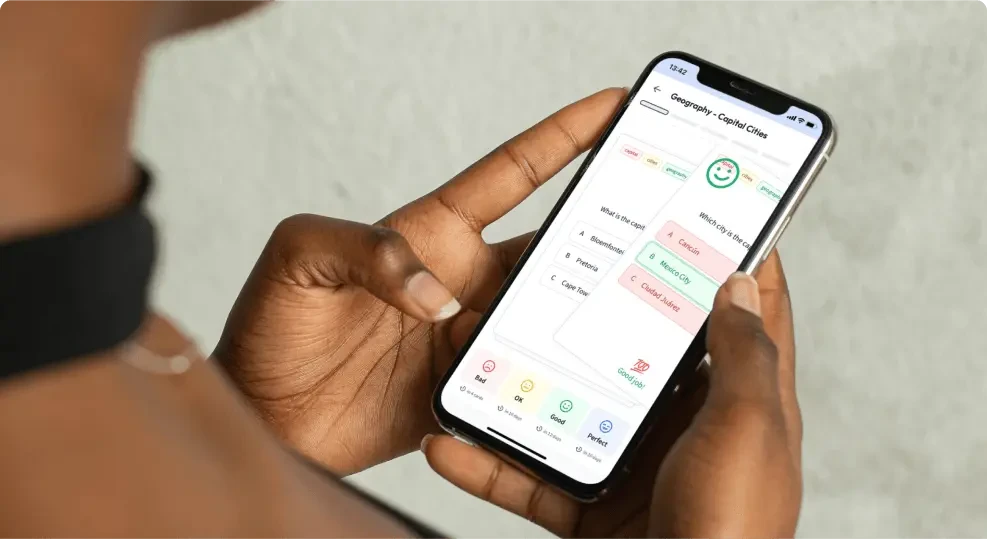
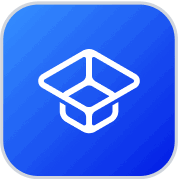
About StudySmarter
StudySmarter is a globally recognized educational technology company, offering a holistic learning platform designed for students of all ages and educational levels. Our platform provides learning support for a wide range of subjects, including STEM, Social Sciences, and Languages and also helps students to successfully master various tests and exams worldwide, such as GCSE, A Level, SAT, ACT, Abitur, and more. We offer an extensive library of learning materials, including interactive flashcards, comprehensive textbook solutions, and detailed explanations. The cutting-edge technology and tools we provide help students create their own learning materials. StudySmarter’s content is not only expert-verified but also regularly updated to ensure accuracy and relevance.
Learn more