Definition of the Doppler Effect in Light
The Doppler effect in light is the phenomenon that is similar to the Doppler effect in sound, namely that the observed frequency of a wave changes when things are moving in the system we are looking at.
The Doppler effect in light is the change in the observed frequency of light caused by the relative movement between the emitter and the observer of the light.
In other words, the emitter will measure the frequency of the light wave to be different than the observer measures it to be. The Doppler effect in light is also called the relativistic Doppler effect.
Difference between Doppler Effect in Light and Sound
There is a difference between the Doppler effect in light and that in sound because sound waves will travel in some medium (e.g. air) which will determine their speed. Light waves do not require a medium to propagate and hence can move through a vacuum. Thus, we have one variable more to consider in the case of sound. The following example best illustrates the difference between the Doppler effect in light and sound.
Suppose the speed between the emitter and the observer is zero. Then in the case of light, there is no Doppler effect. However, if the emitter and the observer are both jet planes, where the emitter is flying behind the observer at the same supersonic speed, we have a whole different situation. The emitter can emit a sound, but the speed of sound is smaller than the speed of the observer! This means that there are no sound waves ahead of the emitter: they all trail behind the emitter, even though the sound waves are propagating in the same direction as the emitter is traveling in. In turn, this means that the sound will never even reach the observer who is in front of the emitter. This effect is demonstrated in the animation below where the emitter clearly outruns the sound waves so there are no sound waves in front of it.
This specific effect can't even happen in the case of light because emitters and observers can never go faster than the speed of light: there will always be light waves in front of the emitter and light waves will always be able to reach the observer.
This specific Doppler effect is possible with sound but not with light, Wikimedia Commons CC BY-SA 3.0.
From the example above, we see that there are not only quantitative differences between the Doppler effect in light and sound but also qualitative differences in some circumstances. The speed of the objects with respect to the medium in the case of sound is essential, while there is no medium at all for light waves. In this sense, the Doppler effect in light is simpler than the Doppler effect in sound.
The Formula of the Doppler Effect in Light
The Doppler effect in light all has to do with the speed of light, particularly the speed between the emitter and the observer relative to the speed of light. Thus, if we call the speed of light \(c\) (as everyone does) and the speed between the observer and the emitter \(v\), then we can define the speed between the observer and the emitter as a fraction of the speed of light as
\[\beta=\frac{v}{c}.\]
This speed is positive if the observer and the emitter move away from each other (which is logical because their distance is increasing) and negative if they move toward each other.
We can now formulate the formula of the Doppler effect in light. This formula relates the frequency that the observer observes, \(f_{r}\), to the frequency that the emitter emits, \(f_{e}\), using the relative speed between the emitter and the observer of the light. The formula is
\[f_\text{r}=\sqrt{\frac{1-\beta}{1+\beta}}f_\text{e}.\]
Let’s see if this is a logical formula. Firstly, we see that the relative frequency difference is only affected by the speed between the emitter and the observer. This makes sense because there aren’t any other quantities of interest in this situation.
Secondly, we want to check the formula qualitatively. If the emitter and the observer are moving toward each other, then \(\beta\) is negative, so \(f_\text{r}>f_\text{e}\). This is correct: The observer measures a higher frequency than the emitter just like the Doppler effect in sound. We do the same check for the situation in which the emitter and the observer are moving away from each other, and we conclude that this formula is indeed qualitatively correct. One more check could be that if the observer and emitter have zero relative speed, then \(\beta =0\) and they agree on the frequency of the light signal.
It turns out that for low speeds relative to the speed of light, we get the following simple approximation:
\[\frac{\Delta f}{f_\text{e}}\simeq \frac{v}{c},\]
where we introduced the frequency difference \(\Delta f=f_\text{e}-f_\text{r}\). This is actually the exact result for the Doppler effect for sound waves (but then where \(c\) is the speed of sound). So for low relative speeds, the Doppler effect in light is very similar to the Doppler effect in sound.
Redshift and Blueshift in the Doppler Effect in Light
Remember that the frequency of light in the visible spectrum determines its color. The lower frequencies are red and the higher frequencies are blue. The difference in the observed frequency of a visible light wave between the emitter and the observer then means that the emitter and the observer of the light wave both see different colors if they have a speed relative to each other!
If the observer measures a lower frequency than the emitter, we speak of a redshift of the light. If the observer measures a higher frequency than the emitter, we talk about a blueshift of the light. We say that the light is redshifted or blueshifted. In general, a redshift or a blueshift is called a Doppler shift.
These terms are generalizations of the color change that would occur in the visible spectrum of light, so these definitions should be easy to remember if you just remember that red light has a lower frequency than blue light.
We can rephrase this as a blueshift being a situation in which \(\Delta f<0\) and a redshift being a situation in which \(\Delta f>0\). Only when \(\Delta f=0\) is there no Doppler shift at all.
If the emitter and observer are moving toward each other, the light is blueshifted, and if the emitter and observer are moving away from each other, the light is redshifted. We show an animation of Doppler shifts below, where a yellow ball (the emitter) appears to change color depending on its speed relative to us (the observer).
Doppler shift of the light emitted by a yellow ball, Wikimedia Commons CC BY-SA 3.0.
We see that the yellow ball undergoes a blueshift when it is moving toward us: the yellow gets shifted toward a bluer color, which in this case is green. When the yellow ball moves away from us, it undergoes a redshift: the yellow gets shifted toward a redder color, orange.
Examples of the Doppler effect in light
A Doppler shift takes place for every relative speed between an emitter and observer of light. However, from the formula above we can see that the frequency change of light due to the Doppler effect only starts to be noticeable when the speed between the emitter and the observer is quite large compared to the speed of light, i.e. when \(\beta\) is not very small.
When you look at a blue car driving away from you, the light it ‘emits’ (reflected sunlight) is slightly redshifted. However, the car’s speed is so small relative to the speed of light that the redshift is not noticeable, so you do not see the color of the car change at all. However, technological equipment can see such small frequency differences. A speed camera emits a light wave of which it knows its frequency, the light wave will reflect off of a car, and the reflected light wave will be Doppler shifted according to the car’s speed. The size of the Doppler shift tells the speed camera the speed of the car, and voilà, we have a working speed camera! See the picture below for a schematic view of the use of the Doppler effect by a speed camera. Note that the difference in wavelength is very exaggerated to show the point.
Speed camera sending a certain frequency of light and receiving another frequency of light. In this case, the car is moving away from the speed camera so the observed light will be redshifted, StudySmarter Originals
Because the universe is expanding, on large scales, everything is moving away from everything. The further away a star is, the faster it’s moving away from the Earth, and thus the more redshifted its emitted light is when it reaches our eyes or our observatories. This can be significant because the relative speed of such a star is pretty large, so observatories need to account for this difference, or they can use the Doppler shift to figure out how far away the star is if they know what frequency of light it emits.
This is also the reason why the sky is mostly dark at night. There is a star in every direction you can look, but most of the stars will be so far away that their light is Doppler shifted entirely into the non-visible spectrum of light.
Doppler Effect for Light - Key takeaways
The Doppler effect in light is the change in the observed frequency of light caused by the relative movement between the emitter and the observer of the light.
The speed of the objects with respect to the medium in the case of sound is essential, while there is no medium at all for light waves.
The formula of the Doppler effect in light is \(f_{r}=\sqrt{\frac{1-\beta}{1+\beta}}f_{e}\).
If the observer measures a lower frequency than the emitter, we speak of a redshift of the light. If the observer measures a higher frequency than the emitter, we speak of a blueshift of the light.
The frequency change of light due to the Doppler effect only starts to be noticeable when the speed between the emitter and the observer is quite large compared to the speed of light.
Speed cameras use the Doppler effect to measure the speed of cars.
The Doppler effect can be very noticeable for distant stars because of the expansion of the universe.
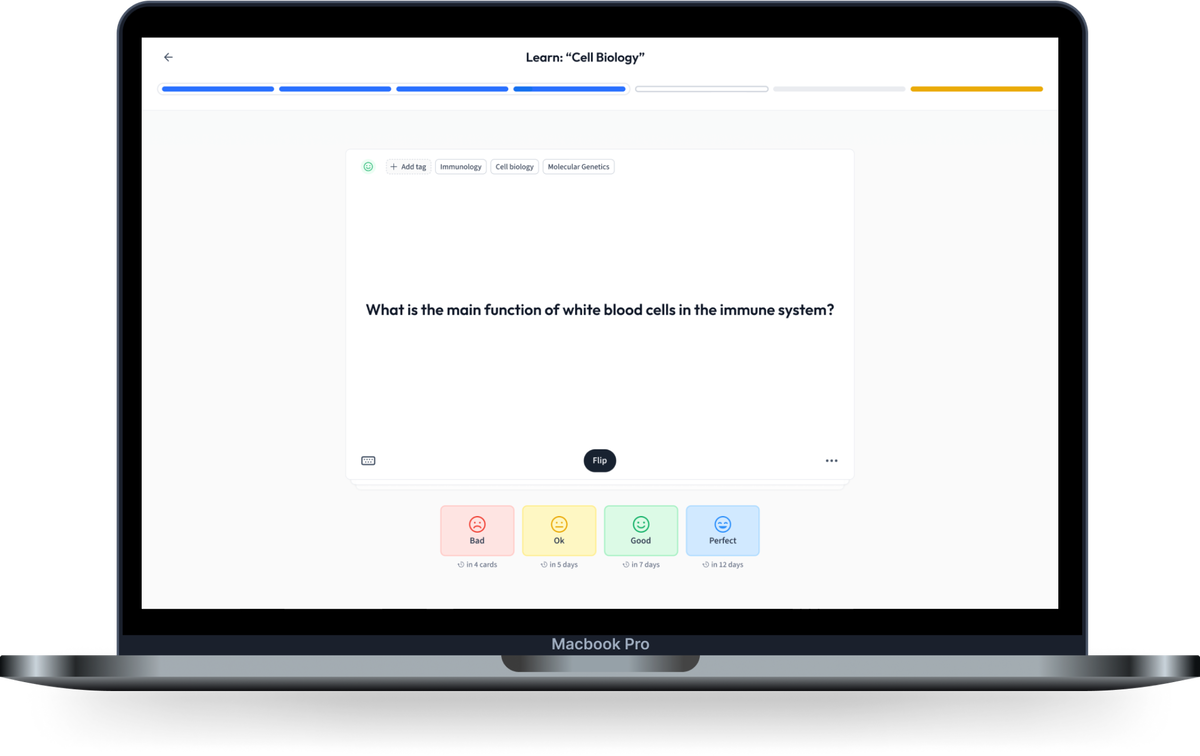
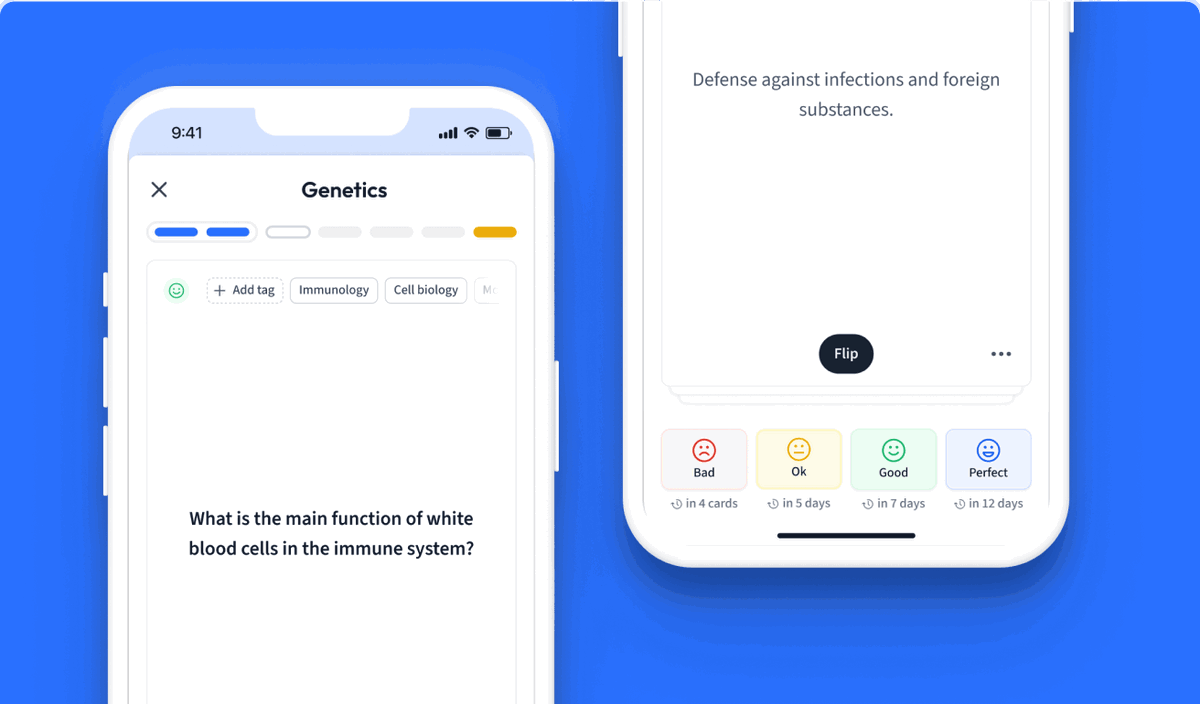
Learn with 15 Doppler Effect in Light flashcards in the free StudySmarter app
We have 14,000 flashcards about Dynamic Landscapes.
Already have an account? Log in
Frequently Asked Questions about Doppler Effect in Light
What is Doppler effect in light?
The Doppler effect in light is the change in the observed frequency of light caused by the relative movement between the emitter and the observer of the light. That is, the emitter will measure the frequency of the light wave to be different than the observer measures it to be.
What is an example of the Doppler effect with light?
An example of the Doppler effect with light is that speed cameras use it to measure the speed of passing cars.
What does the Doppler effect measure?
The Doppler effect can be used to measure the relative speed between the emitter and the observer of a wave.
How is the Doppler effect different for light?
There is a difference between the Doppler effect in light and sound because there is a medium for sound waves that can travel at its own speed. Thus, we have one variable less to take into account in the case of light.
What is the formula for Doppler effect in light?
The formula describing the Doppler effect in light is f_r=((c-v)/(c+v))^(1/2)*f_e, where f_r and f_e are the frequencies measured by the observer and the emitter of the lightwave, respectively, v is the relative velocity between the observer and the emitter, and c is the speed of light.
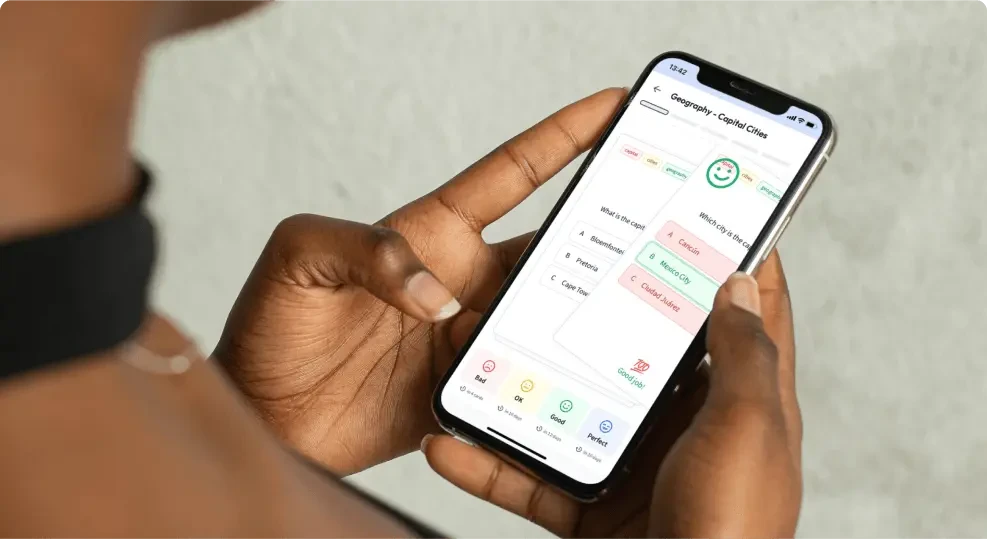
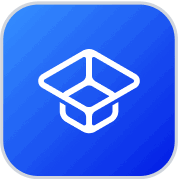
About StudySmarter
StudySmarter is a globally recognized educational technology company, offering a holistic learning platform designed for students of all ages and educational levels. Our platform provides learning support for a wide range of subjects, including STEM, Social Sciences, and Languages and also helps students to successfully master various tests and exams worldwide, such as GCSE, A Level, SAT, ACT, Abitur, and more. We offer an extensive library of learning materials, including interactive flashcards, comprehensive textbook solutions, and detailed explanations. The cutting-edge technology and tools we provide help students create their own learning materials. StudySmarter’s content is not only expert-verified but also regularly updated to ensure accuracy and relevance.
Learn more