Understanding Waves in Coastal Geography
Waves are a fundamental aspect of coastal geography, shaping shorelines and influencing marine and terrestrial ecosystems. They play a critical role in processes such as erosion, transportation, and deposition of sediments. For students aiming to study geography at a university level, a detailed understanding of wave dynamics is essential.
Wave Definition and Basics
Wave Definition: A wave is a disturbance that transfers energy through a medium, often resulting in the rhythmic rising and falling of the surface in bodies of water.
When discussing waves within the context of geography, it is important to comprehend the basics of how waves function. These natural phenomena are more than just rolling water; they are the movement of energy through the water, which in turn affects coastal landscapes dramatically. Wave energy originates from wind, which is why understanding weather patterns is also a key aspect of coastal geography.
Explaining Wave Formation
The formation of waves is a complex process influenced by factors such as wind speed, duration, and the distance over which the wind blows across the water's surface, known as the fetch. The interaction between the wind and the water's surface causes ripples which can grow into larger waves. This action is described scientifically by the principle of friction: as wind moves across the water surface, it causes the water to move along with it, creating waves.
Wave energy and the resulting wave size are directly correlated to the strength and duration of the wind, as well as the size of the area over which the wind interacts with the water. The transformation of this energy is not only visible on the surface but also affects the water column, sometimes down to significant depths. Specialised terminology also plays a part in the study of wave formation. For instance, capillary waves, which are small ripples, often precede larger waves called gravity waves, which are created by the force of gravity pulling back on the water displaced by wind energy.
Characteristics of Waves in Geography
Waves hold various characteristics that define their physical properties and the influence they have on the coastline. These include wave height, wavelength, period, speed, and frequency, all of which geographers use to measure and predict wave behavior. Understanding these characteristics is vital for predicting the impact of waves on coastal erosion and for designing coastal defences.
Wave Characteristics in Geography: The attributes of waves, including height, length, period, frequency, and speed, which describe their physical properties and impacts on coastal environments.
Measuring the Amplitude of Waves
The amplitude of a wave is a measure of its magnitude and is typically defined as the distance from the still water level to the crest of a wave. It is one-half of the wave's height and can be calculated using the formula: \[Amplitude = \frac{Wave Height}{2}\]
Geographers measure the amplitude to estimate the wave's energy and potential impact on the coastline. It's important to note that the energy of a wave increases with the square of the amplitude; therefore, slight increases in amplitude can significantly affect the wave's power.
Types of Waves and Their Impact on Coasts
The coastal landscape is continuously modelled and remodelled by various types of waves, which can be broadly categorised as either longitudinal or transverse. These waves carry energy across the ocean's surface, often culminating in powerful interactions with coastlines that can result in significant geographical phenomena like erosion and deposition. Comprehending the nature of these waves and their impact is fundamental for predicting and managing coastal change.
Differentiating Longitudinal and Transverse Waves
Longitudinal and transverse waves differ fundamentally in the direction of energy transfer relative to the motion of the medium through which the wave moves. Longitudinal waves are characterised by the oscillation of particles in the medium in the same direction as the wave travels. Conversely, transverse waves involve oscillations perpendicular to the direction of the wave's advance.
Longitudinal and Transverse Waves: The two main types of wave motion where the former involves particles oscillating parallel to the direction of energy transfer, while the latter has particles moving perpendicular to the direction of the wave.
- Longitudinal Waves: Such waves in geology typically manifest as seismic P-waves, which surge through the Earth during earthquakes. Their compression and rarefaction phases alternate, effectively pushing and pulling on the material's particles as they propagate. This type of wave is also responsible for the sound waves heard every day.
- Transverse Waves: Striking examples of transverse waves are light waves and the ripples on a water surface. In coastal geography, when the wind blows, waves formed on the ocean's surface oscillate water particles in an up-and-down motion, characteristic of transverse waves.
To visualise the difference between these two types of waves, imagine a slinky. When compressing and releasing one end of the slinky, the coils move back and forth in a straight line, representing a longitudinal wave. If the same slinky is fixed at one end and the other end is moved up and down, the resulting motion through the slinky will create peaks and troughs, demonstrating a transverse wave.
Wave Refraction Geography and Coastal Shaping
Wave refraction is a pivotal process in coastal geography, accounting for the bending of waves as they approach shallow water near the shoreline. This phenomenon is influentially involved in shaping the coastline by differentially eroding headlands and depositing sediments in bays and inlets. Understanding the principles of wave refraction and its geographical consequences is critical in coastal management and conservation.
Wave Refraction Geography: It is the study of how waves bend as they hit the coastline at an angle, causing uneven distribution of energy and significantly influencing coastal shaping through erosion and deposition.
Wave refraction occurs due to the decrease in wave velocity as water depth decreases. When waves approach a coast at an angle, the part of the wave in shallower water slows down more than the part still in the deeper water, causing the wave to bend and align more closely with the coastline.
Wave Velocity = Frequency × Wavelength
As a result of refraction, wave energy is concentrated on headlands and dispersed within bays. This concentrates erosion on the more prominent parts of the coastline while encouraging deposition in the more recessed areas. Geographers use this knowledge to anticipate changes in coastal landscapes and develop strategies to mitigate negative impacts of coastal erosion.
The Role of Wave Refraction in Erosion and Deposition
Wave refraction plays a dual role in coastal processes. It exacerbates erosion on protruding coastlines while simultaneously fostering deposition in sheltered areas. The bending of wave crests as they engage with the shoreline causes energy to be distributed unevenly along the coast. As a consequence, areas experiencing greater wave energy will suffer more intense erosion, and areas where energy is dispersed will become zones of sediment accumulation.
The erosion of headlands forms distinct coastal features like cliffs, wave-cut platforms, and sea arches, while bays may fill with sediments to form beaches and spits. Over time, wave refraction can lead to the straightening of irregular coastlines, with headlands being eroded back and bays infilled with sediments. Tools such as GIS (Geographic Information Systems) and computer simulations are routinely utilised by geographers to predict and visualise these coastal transformations over time.
How Wave Energy Influences Erosion Patterns
The energy associated with a wave is a determining factor in coastal erosion patterns. Waves with higher energy have a greater capability to transport and deposit beach material, reconfiguring the coastal landscape. This energy is derived from wind, which imparts motion to the water's surface, and is influenced by factors such as wind speed, direction, and duration, as well as fetch – the distance over which the wind blows across open water.
Amplitude of Waves: The measure from the still water level to the crest, representing half the wave height, with larger amplitudes indicating greater energy transfer and potential for erosion.
The formula for wave energy indicates that it is proportionate to the square of the wave amplitude: \[Wave Energy \propto Amplitude^2\]
Therefore, a small increase in amplitude results in a considerable increase in energy, which intensifies the wave's ability to erode the coastline. High-energy waves can carry large rocks and sediments, sculpting the landscape aggressively. Understanding these dynamics allows geographers to assess erosion risks and devise coastal defence measures.
Waves with long wavelengths and high frequency, such as tsunamis generated by undersea earthquakes, can have devastating impacts on coasts due to their immense energy and amplitude.
Investigating Wave Erosion Features
Wave erosion features are integral elements of coastal geography that reveal the dynamic interactions between the ocean's energy and the land it encounters. Examining these natural structures provides insight into historical wave activity and serves as a gauge for future coastal changes. For A Level geography students, understanding these features helps to grasp the complexities of coastal processes and the power of natural forces in shaping our planet.
Identifying Common Wave Erosion Landforms
Common wave erosion landforms such as headlands, bays, cliffs, arches, stacks, and wave-cut platforms showcase the amazing sculpting power of wave action over time. Through the relentless force of wave erosion, these features are formed and then transformed, providing a living laboratory for the study of coastal geomorphology. The nature and rate of erosion leading to these landforms depend on several factors, including the rock type, wave energy, coastal geometry, and sea level changes. By identifying and analysing these structures, one can infer not only past climatic conditions but also predict how current and future environmental factors may reshape coastlines.
Cliffs and Wave-Cut Platforms: A Result of Erosion
Among the most visible wave erosion features are cliffs and wave-cut platforms. Cliffs are steep rock faces rising near vertically from the sea, formed by the undercutting activity of waves. The base of a cliff is continually eroded by wave action, leading to an overhang of unsupported rock which eventually collapses. As erosion progresses, it leaves behind a relatively flat, often horizontal area at the base known as a wave-cut platform.
Wave-Cut Platform: A flat, bench-like surface lying below cliffs, created by the abrasion of waves against the base of cliffs, slowly cutting back the land.
An exemplary depiction of a wave-cut platform can be found on the coastline of Dorset, UK, where the iconic Lulworth Cove presents a clear demarcation of a cliff leading to a well-defined platform extended into the sea.
Wave-cut platforms are rife with tidal pools and other features that support diverse marine ecosystems. They change dramatically over time, with their surfaces telling a story of past wave conditions, biological activity, and historical sea levels. Their formation can even influence the development of other geological structures, such as caves, arches, and sea stacks, by altering wave energy concentration and directional flow.
The Process of Erosion by Waves
The process of erosion by waves is one of the most powerful forces shaping coastal landscapes. It is a relentless, cyclic process where the energy of waves collides with the shore, abrading and breaking down rock into sediment. This process is influenced by factors like the geology of the coastline, wave energy, tide levels, and the presence of protective features such as beaches and vegetation. Over time, the repetition of these forces can lead to the dramatic alteration of coastal geography, carving out bays, and creating new landforms such as cliffs and wave-cut platforms.
A critical sub-process within wave erosion is hydraulic action, where the force of water and trapped air in cracks and crevices of rock formations can lead to material dislodgment. Additionally, abrasion or 'corrasion', the 'sandpapering' effect of sediment carried by waves against rocks, contributes to the wearing down of coastal features. Moreover, waves can dissolve water-soluble minerals in the rocks through a process known as solution or corrosion, further enhancing erosion.
The Connection Between Wave Energy and Erosion Rate
There is a direct correlation between the energy carried by waves and the rate of coastal erosion. High-energy waves, often associated with storm events, have a greater capacity to erode landforms rapidly. The energy of a wave is classically related to its amplitude and period (the time between successive crests or troughs), with the energy per unit area of the wave being proportional to the square of the amplitude, as shown by the equation: \[Energy ∝ \frac{1}{2} \rho g A^2\]
where Energy is the energy per unit area, \( \rho \) is the water density, g is the acceleration due to gravity, and A is the amplitude of the wave.
Wave Energy: A key driver of coastal erosion, defined as the amount of energy transported by a wave as it travels across the ocean's surface.
The erosion rate is also significantly determined by the geological composition of the coastline; softer materials like clay and sand erode faster than harder materials such as granite.
For instance, during the winter storms, coastlines exposed to powerful oceanic waves, such as those facing the Atlantic in southwest England, undergo significant and rapid erosion. These high-energy waves can remove large amounts of material, dramatically reshaping the coastline.
Understanding the connection between wave energy and erosion rate is crucial for coastal management. Strategies such as the construction of sea walls, groynes, and the use of beach nourishment must account for the varying wave energies to be effective. Additionally, this knowledge informs the placement of infrastructure, guiding policymakers to build at safe distances from potentially erodible coastlines.
The Influence of Waves on Coastal Development
Coastal development is significantly shaped by wave action, which can create, alter, and sometimes destroy human-made structures and natural landscapes. The energy of waves, influenced by factors like wind strength, fetch, and seabed topography, can dictate the extent and form of coastal development. By shaping beaches, eroding cliffs, and sometimes providing opportunities for recreational activities, waves have a profound effect on how coastal areas are utilised and inhabited.
Waves' Impact on Human Activities Along Coasts
The interplay between waves and human activities along coastal regions is both beneficial and challenging. Waves shape beaches and coastal landscapes, offering opportunities for tourism and recreation. However, the constant presence of wave action also poses risks to coastal infrastructure, necessitating careful planning and management.The strength and direction of waves can considerably impact marine navigation, fishing, and the construction of marine structures like harbours and jetties. These activities depend on calm water conditions, which can be disrupted by strong wave action leading to hazardous maritime conditions, interference with fishing activities, and damage to coastal infrastructure.Moreover, powerful storm waves can lead to flooding and erosion, threatening coastal settlements and leading to costly damage and necessary relocation. The growth of coastal resorts and recreational areas is thus directly influenced by the nature of the waves, with calm bays often becoming hotspots for development while rough waters may discourage such investments.In areas of high wave energy, developing sea wall defences, breakwaters, and groynes are essential to protect human habitats and maintain sustainable economic activity. These structures themselves, however, can modify wave patterns, potentially leading to unintended consequences such as increased erosion elsewhere - a phenomenon that must be studied and predicted by coastal engineers and geographers.
For example, the construction of sea walls in one area can lead to the increased erosion of down-drift beaches, as seen in parts of the UK's coastline. This is due to the interference with the natural process of longshore drift, which is pivotal in sand and sediment distribution along the coast.
Famously, surfing activities are centred around coasts with consistent and sizeable waves, turning such places into vibrant hubs for sport and culture.
Conservation of Coastal Areas Affected by Waves
Conservation efforts in coastal areas are deeply intertwined with the understanding of waves and their impacts. The goal is to preserve natural landscapes and biodiversity while mitigating the threats posed by wave-induced erosion and extreme weather events such as storm surges. Conservation strategies include both hard engineering solutions like the construction of artificial defences and soft engineering methods such as the restoration of sand dunes and mangrove planting.When devising conservation strategies, it is crucial to consider the long-term effects of interventions on natural processes. For instance, hard structures may provide immediate protection but can alter sediment transport and potentially harm marine ecosystems. In contrast, soft engineering solutions aim to work with nature, enhancing the natural resilience of coastlines against wave action without disrupting ecological balance.The preservation of beaches through controlled beach nourishment schemes is a common practice, particularly in tourist-reliant regions. This involves the replenishment of beach sediment, effectively absorbing wave energy and reducing erosion.Environmental impact assessments are of utmost importance in these conservation efforts. They examine the potential consequences of any planned development or conservation project on coastal environments. Conservationists often collaborate with geographers and oceanographers to understand wave dynamics better and design interventions that can withstand the test of time.
One impactful conservation method is the use of living shorelines, which incorporate natural elements like oyster reefs and native vegetation to stabilise the coast. These sustainable approaches are designed to enhance the coastal ecosystem's services, such as water filtration and habitat provision while attenuating wave energy. This not only preserves the shoreline but also promotes biodiversity and water quality improvements.
The development and conservation of coral reefs are vital as they act as natural breakwaters, dissolving wave energy and protecting shorelines from erosion.
Waves and Coastal Management Strategies
Comprehending the intricacies of waves is crucial for effective coastal management, as they are a dominant force in shaping the world's shorelines. Coastal management strategies must account for the natural dynamics of waves, including their formation, characteristics, and the impact they have on erosion and deposition processes. These strategies are designed to protect, restore, and sustainably develop coastal regions by mitigating adverse effects of wave action and adapting to changing environmental conditions.
Natural and Human-Induced Changes to Wave Patterns
Wave patterns are subject to change due to both natural processes and human activities. Naturally, factors such as ocean currents, storms, tidal effects, and seismic events can alter the dynamics of wave formation and behaviour. For instance, storm systems can generate larger, more energetic waves, while undersea earthquakes can lead to tsunami waves with long wavelengths and periods.Human-induced changes stem from alterations to coastal geomorphology through construction, dredging, and the addition of coastal defences. Infrastructure such as breakwaters, groynes, and seawalls can alter wave patterns, potentially leading to unexpected issues like increased erosion downstream or changes in sediment transport.Beach nourishment, often used to counteract erosion, can also affect local wave conditions by changing beach profile and, subsequently, the way waves break and dissipate energy. Therefore, both natural and anthropogenic factors must be understood and considered when developing coastal management strategies to ensure the long-term stability and health of coastal ecosystems.
It is important to study historical data and predictive models to comprehend the potential long-term impacts of these changes. Advanced tools such as satellite imagery, aerial drones, and wave simulation software play an integral part in detecting and quantifying these changes. With the looming challenge of climate change, sea-level rise, and increased storm frequency and intensity, the understanding of wave patterns becomes all the more poignant for future-proofing coastal areas against natural disasters and preserving their economic and environmental value.
Adaptive Measures to Mitigate Wave-Induced Erosion
Adaptive measures to mitigate wave-induced erosion are crucial to managing and sustaining coastal environments. These measures include a combination of hard engineering and soft engineering strategies, each with distinct approaches and implications.Hard engineering involves the construction of physical structures like seawalls, breakwaters, and revetments. These are designed to absorb or deflect the energy of incoming waves, protecting the coastline from the immediate effects of erosion. However, they can be expensive, visually intrusive, and may have negative environmental impacts by altering natural sediment transport.Soft engineering, conversely, involves more environmentally friendly alternatives that work with natural processes. Examples include beach nourishment, dune stabilisation, and the planting of vegetation to secure soil. These methods aim to increase the coastline's natural resilience against wave action and are often more sustainable in the long term.Implementing these adaptive measures requires careful planning and continuous monitoring to ensure they are effectively reducing erosion without causing detrimental effects elsewhere. One must also consider the balance between protecting existing infrastructures and allowing natural coastal processes to occur, maintaining a dynamic equilibrium of the shoreline.
An excellent example of a mixed strategy is the use of rock armour (also known as riprap) along with dune restoration. The rock armour provides immediate protection against strong waves, while the dune restoration, over time, develops a natural barrier to dissipation of wave energy.
Increasingly, coastal managers are looking towards 'living shoreline' solutions that incorporate natural elements such as salt marshes, oyster reefs, and mangrove forests. These habitats not only reduce wave energy but also provide significant ecological benefits, including enhanced biodiversity, water filtration, and carbon sequestration. Adopting adaptive measures requires an interdisciplinary approach, incorporating oceanography, engineering, and environmental science to create strategies that are resilient in the face of both natural dynamics and human needs.
The concept of 'managed retreat' is also emerging as a viable adaptive measure, where areas particularly vulnerable to erosion are allowed to evolve naturally, with human activities and structures being strategically relocated or removed.
Waves - Key takeaways
- Wave definition: A wave is a disturbance that transfers energy through a medium, often resulting in the rhythmic rising and falling of the surface in bodies of water.
- Wave formation: Influenced by wind speed, duration, and fetch, waves begin as ripples and can grow into larger waves; larger waves are formed from the energy displaced by wind interacting with water.
- Characteristics of waves in geography: These include height, length, period, frequency, and speed, which are important for understanding wave behavior and coastal erosion.
- Amplitude of waves: Defined as the distance from the still water level to the crest of the wave, it's half the wave height and important for estimating wave energy and potential coastal impact.
- Types of waves: Longitudinal waves involve oscillations in the direction of the wave travel, while transverse waves have oscillations perpendicular to the direction of travel; these waves' interactions with coastlines can lead to significant geographical phenomena like erosion and deposition.
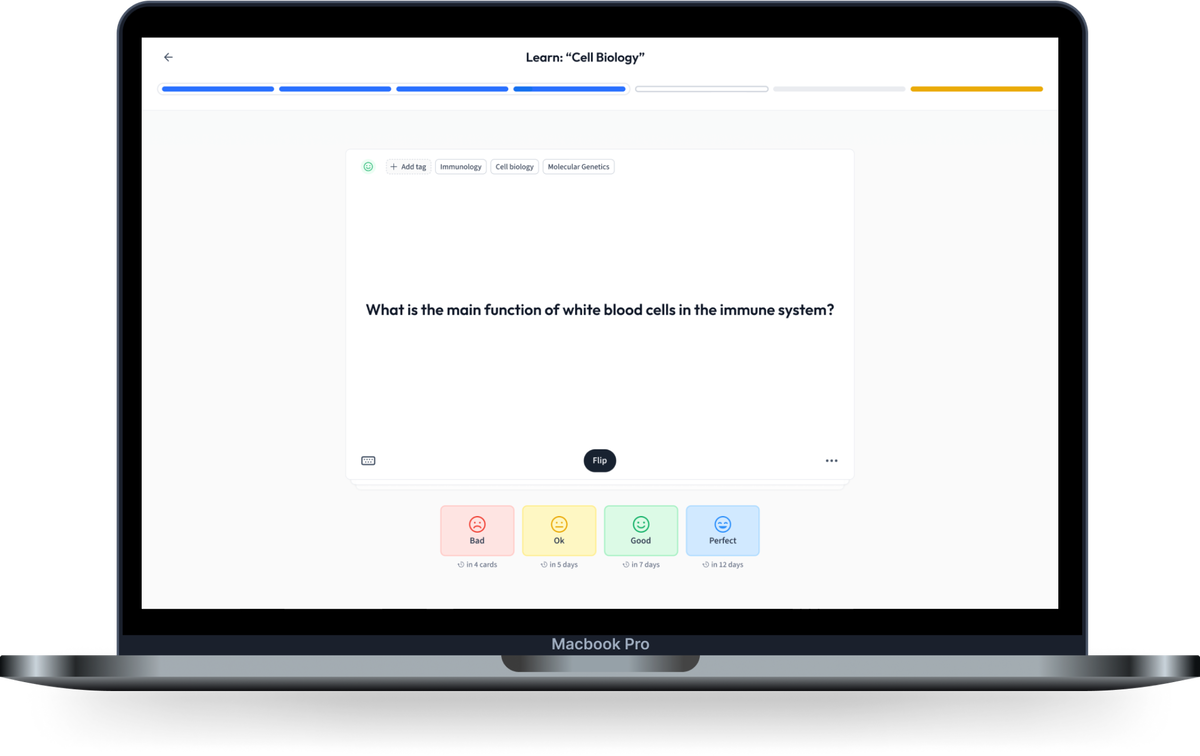
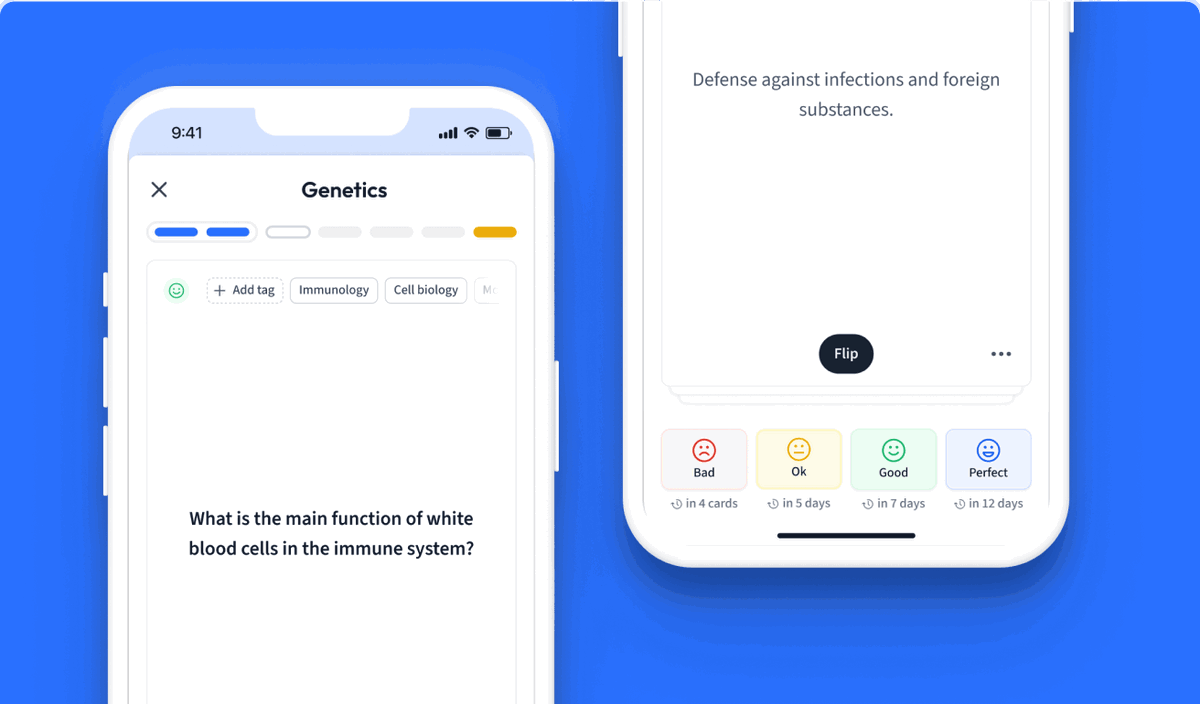
Learn with 29 Waves flashcards in the free StudySmarter app
We have 14,000 flashcards about Dynamic Landscapes.
Already have an account? Log in
Frequently Asked Questions about Waves
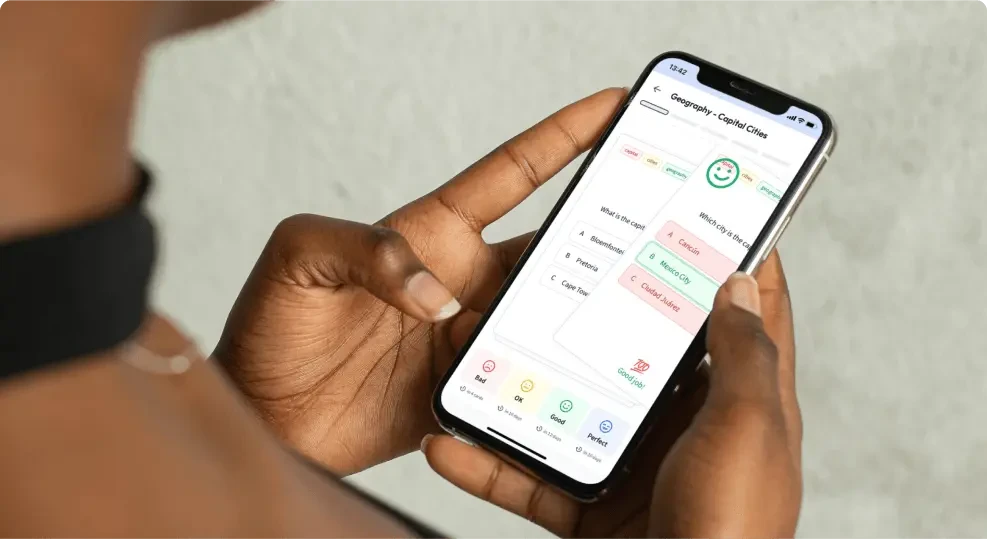
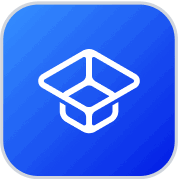
About StudySmarter
StudySmarter is a globally recognized educational technology company, offering a holistic learning platform designed for students of all ages and educational levels. Our platform provides learning support for a wide range of subjects, including STEM, Social Sciences, and Languages and also helps students to successfully master various tests and exams worldwide, such as GCSE, A Level, SAT, ACT, Abitur, and more. We offer an extensive library of learning materials, including interactive flashcards, comprehensive textbook solutions, and detailed explanations. The cutting-edge technology and tools we provide help students create their own learning materials. StudySmarter’s content is not only expert-verified but also regularly updated to ensure accuracy and relevance.
Learn more