What is Photoelectric Effect?
Photoelectricity, or the photoelectric effect, is a fascinating physical phenomenon involving the emission of electrons from a material when it absorbs light. This principle is not only intriguing but forms the bedrock of various modern technologies, including solar panels and digital cameras.
Understanding the Basics of Photoelectricity
At its core, the photoelectric effect is about the conversion of light energy into electrical energy. When light, which is constituted of packets called photons, hits certain materials, it can free electrons from the surface of these materials. This liberation of electrons is what is referred to as the photoelectric effect. But not all lights can trigger this phenomenon - the frequency of the light must be above a certain threshold specific to the material.
Photoelectric Effect: The release of electrons from a material's surface as a result of exposure to electromagnetic radiation, such as light, beyond a certain threshold frequency.
Example: In solar cells, the photoelectric effect is utilised to convert sunlight into electricity. Light photons hit the solar cell, knocking electrons loose and generating an electric current.
- The work function is a crucial concept in understanding photoelectricity. It's the minimum amount of energy needed to remove an electron from the surface of a material.
- The effect also demonstrates quantum mechanics, showing that light has both particle and wave properties, where the photons represent the particle aspect.
Historical Discovery of the Photoelectric Effect
The discovery of the photoelectric effect dates back to the late 19th century, with several physicists contributing to its understanding. However, it was Albert Einstein who, in 1905, provided a groundbreaking explanation of the phenomenon, anchoring it firmly within the realm of quantum mechanics. His work on this subject earned him the Nobel Prize in Physics in 1921.
Einstein proposed that light could be thought of as made up of particles, or "quanta", that could impart energy to electrons. This was a significant leap forward, as it contradicted the classical wave theory of light prevalent at the time. Einstein's work laid the foundation for modern quantum physics and opened up new avenues for technological advancements.
Deep Dive: Prior to Einstein, scientists like Heinrich Hertz and Philipp Lenard had observed the photoelectric effect, but it was Einstein who explained it as a quantised phenomenon, which led to the wider acceptance of quantum theory.
How Photoelectricity Changed Physics
The understanding and application of photoelectricity have revolutionised physics in several ways. It not only proved the dual nature of light but also played a pivotal role in the development of quantum mechanics. The practical applications of the photoelectric effect are vast, ranging from the technology in digital cameras to the functioning of astronomical telescopes.
Moreover, the photoelectric effect has implications in everyday technologies and innovations. For example, it's the principle behind the operation of automatic doors, which use light sensors to detect the presence of an object or person.
Did you know? The speed of an ejected electron in the photoelectric effect is not determined by the intensity of light, but by its frequency; a greater frequency (above the threshold level) means higher kinetic energy for the ejected electron.
The embracing of photoelectricitypaved the way for advancements in multiple fields, including electronics and renewable energy, demonstrating the power of quantum physics to transform the technological landscape. As exploration of the quantum realm continues, the potential applications and understandings of phenomena like the photoelectric effect are bound to expand further.
Photoelectricity Examples
Exploring photoelectricity through practical demonstrations and real-world applications enhances the understanding of how light can be converted into electrical energy. This intriguing phenomenon is not just a cornerstone of modern physics but also a foundation for numerous technologies used in everyday life.
Practical Demonstrations of the Photoelectric Effect
One of the best ways to grasp the concept of photoelectricity is through hands-on experiments that visually demonstrate the effect in action. Below are some illustrative examples:
Example: A classic demonstration involves shining ultraviolet (UV) light on a zinc plate connected to an electroscope. The zinc plate, upon exposure to UV light, emits electrons due to the photoelectric effect, causing the electroscope's leaves to diverge, visually indicating the presence of electrical charge.
Example: Another compelling demonstration uses a photocell (or photoelectric cell) circuit to show how light intensity and frequency affect the current produced. This setup usually incorporates a variable light source, allowing observers to see firsthand the threshold frequency required to initiate electron emission.
These demonstrations not only illustrate the photoelectric effect but also serve as a bridge to understanding more complex quantum phenomena.
- Such experiments highlight the necessity of threshold frequency: light below this frequency, regardless of its intensity, fails to eject electrons.
- They also provide insight into how electron emissions increase with light intensity once the threshold frequency is surpassed, an essential attribute of the photoelectric effect.
Everyday Applications of Photoelectricity
The principles of photoelectricity find their practical applications in various devices and technologies we interact with daily. Here are some examples where the photoelectric effect plays a critical role:
Example: Solar Panels: One of the most well-known applications of photoelectricity is in solar panels, where light photons free electrons in a semiconductor, creating a flow of electric current that can be harnessed as renewable energy.
Example: Automatic Doors: Many automatic door systems use photoelectric sensors. When the beam of light between the emitter and receiver is interrupted by someone approaching the door, the sensor triggers the door to open.
Example: Light Meters: Used in photography, light meters gauge the intensity of light using photoelectric cells. The electrical current generated by these cells varies with light intensity, enabling precise adjustments for optimal exposure.
- These applications underscore the importance of threshold frequency and the behaviour of electrons upon absorbing light energy, demonstrating the real-world impact of the photoelectric effect.
- Furthermore, such technologies exploit the capacity to directly convert light into electrical signals or power, showcasing the photoelectric effect's versatility and efficiency.
Photoelectric Effect Equation
The Photoelectric Effect Equation is a mathematical representation that describes the energy of electrons emitted from a surface as a result of photoelectricity. This equation not only underpins our understanding of the mechanism but also enables the calculation of critical variables such as kinetic energy, frequency, and work function.
Breaking Down the Photoelectric Equation
The equation for the photoelectric effect can be expressed as KE = hf - φ, where KE is the kinetic energy of the emitted electrons, h is Planck's constant, f is the frequency of the incident light, and φ (phi) is the work function of the material.
Kinetic Energy (KE): The energy that an electron possesses due to its motion. Planck's Constant (h): A fundamental constant in quantum mechanics that relates the energy of a photon to its frequency, approximately 6.626 x 10-34 m2kg/s. Frequency (f): The number of waves that pass a certain point in a specified period of time, typically measured in Hertz (Hz). Work Function (φ): The minimum amount of energy required to remove an electron from the surface of a material.
Example: For a material with a work function of 2.0 eV (electronvolts) and light with a frequency of 1.5 x 1015 Hz shining on it, the kinetic energy of the emitted electrons can be calculated using the above equation.
- The equation emphasizes the fundamental principle that the kinetic energy of the ejected electrons depends on the frequency of the incident light and the material's work function.
- It illustrates a direct correlation between the energy of the incoming photons and the energy with which electrons are emitted, minus the binding energy (work function) that kept the electrons bound to the material.
How to Calculate Energy in Photoelectricity
Calculating energy in the context of photoelectricity involves understanding how to apply the photoelectric effect equation to various scenarios. This calculation allows physicists and engineers to design and optimize devices like photovoltaic cells and sensors.
Remember to convert the work function from electronvolts (eV) to Joules (J) when using it in calculations with Planck's constant, as they need to be in compatible units.
Variable | Description | Unit |
KE | Kinetic Energy of Electrons | Joules (J) |
h | Planck's Constant | m2kg/s |
f | Frequency of Incident Light | Hertz (Hz) |
φ | Work Function | Joules (J) or Electronvolts (eV) |
Example: To calculate the kinetic energy of the emitted electrons in Joules, first ensure all units are consistent. If the work function is given in eV, it must be converted to Joules using the conversion factor 1 eV = 1.602 x 10-19 J. After conversion, apply the values to the equation KE = hf - φ.
Deep Dive: The photoelectric effect equation deftly encapsulates the quantum relationship between light and matter. Its development marked a pivotal moment in physics, laying the groundwork for quantum mechanics and challenging classical notions of light and energy. It serves as a tangible application of quantum principles to real-world phenomena and technologies.
Photoelectric Effect Applications
Photoelectric Effect Applications extend far beyond the confines of traditional physics textbooks, infiltrating various sectors of modern technology and everyday devices. This phenomenon, which underlines the conversion of light into electrical energy, has paved the way for innovations spanning from green energy solutions to advanced imaging devices.
Einstein and the Photoelectric Effect
Albert Einstein's contribution to our understanding of the photoelectric effect catalysed a paradigm shift in the field of physics. His groundbreaking paper, presented in 1905, introduced the concept of light quantisation, suggesting that light could be absorbed or emitted in discrete packets known as photons.
Photoelectric Effect (Einstein’s Perspective): The phenomenon where electrons are emitted from materials (metals and non-metallic solids, liquids, or gases) upon exposure to light above a certain threshold frequency, directly supporting the quantum theory of light.
Example: Einstein's equation for the photoelectric effect, E = hf - φ, where E represents the electron's energy, hf the energy of the photon, and φ the work function of the material, precisely described how photons eject electrons from a material.
Einstein's work on the photoelectric effect not only earned him the Nobel Prize in Physics in 1921 but also fundamentally supported the dawning era of quantum mechanics.
Modern Uses of Photoelectricity in Technology
Today, the principles of photoelectricity are harnessed in numerous technological applications, demonstrating the wide-ranging impact of this quantum phenomenon. Here are some key areas where photoelectricity plays a pivotal role:
Example: Solar Power Generation: Perhaps the most recognised application, solar panels convert sunlight into electricity using the photoelectric effect, illustrating a sustainable path towards energy generation.
Example: Safety Sensors: In industrial settings, photoelectric sensors are used extensively for automating processes. These sensors, detecting the presence or absence of objects through light beams, optimise productivity and safety.
- Optical Communication: The transmission of data through light waves, including fibre-optic communication, relies on the photoelectric effect to convert optical signals into electrical ones, facilitating high-speed internet and telecommunications.
- Photography and Imaging: Digital cameras and night vision devices use photoelectric sensors to capture images. These sensors translate varying light intensities into electronic signals, creating the digital image.
Deep Dive: The intersection of photoelectricity with nanotechnology is spearheading the development of next-generation photovoltaic cells. These advanced solar cells not only promise higher efficiencies but also flexible and lightweight designs, opening new avenues for integrating solar power into everyday objects and buildings.
Photoelectricity - Key takeaways
- Photoelectric Effect: Emission of electrons from a material's surface when exposed to light above a certain threshold frequency.
- Work Function (φ): The minimum energy needed to remove an electron from a material's surface, a key factor in the photoelectric effect.
- Photoelectric Effect Equation (Einstein's Equation): KE = hf - φ, where KE is the kinetic energy of emitted electrons, h is Planck's constant, f is the frequency of the incident light.
- Einstein's Contribution: Awarded the Nobel Prize in Physics for explaining the photoelectric effect, which supports the quantum theory of light.
- Applications of Photoelectricity: Found in daily technologies like solar panels, automatic doors, and light meters, and pivotal in advancing fiber-optic communication and digital imaging.
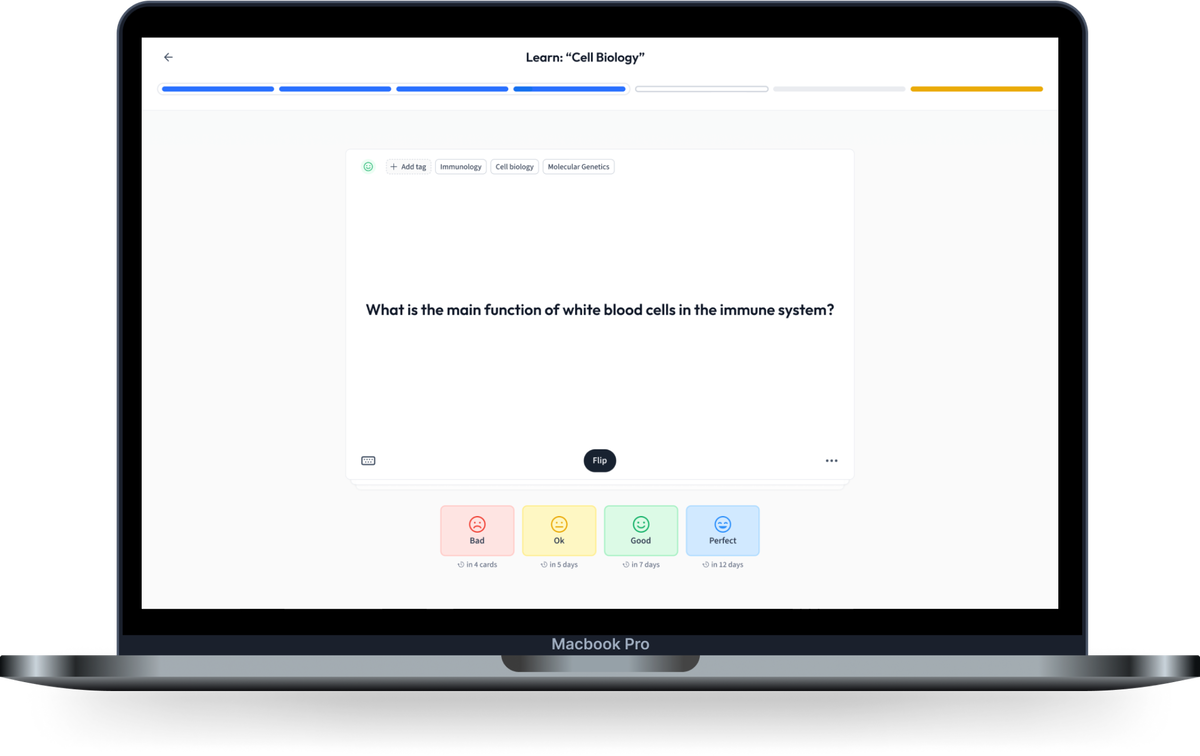
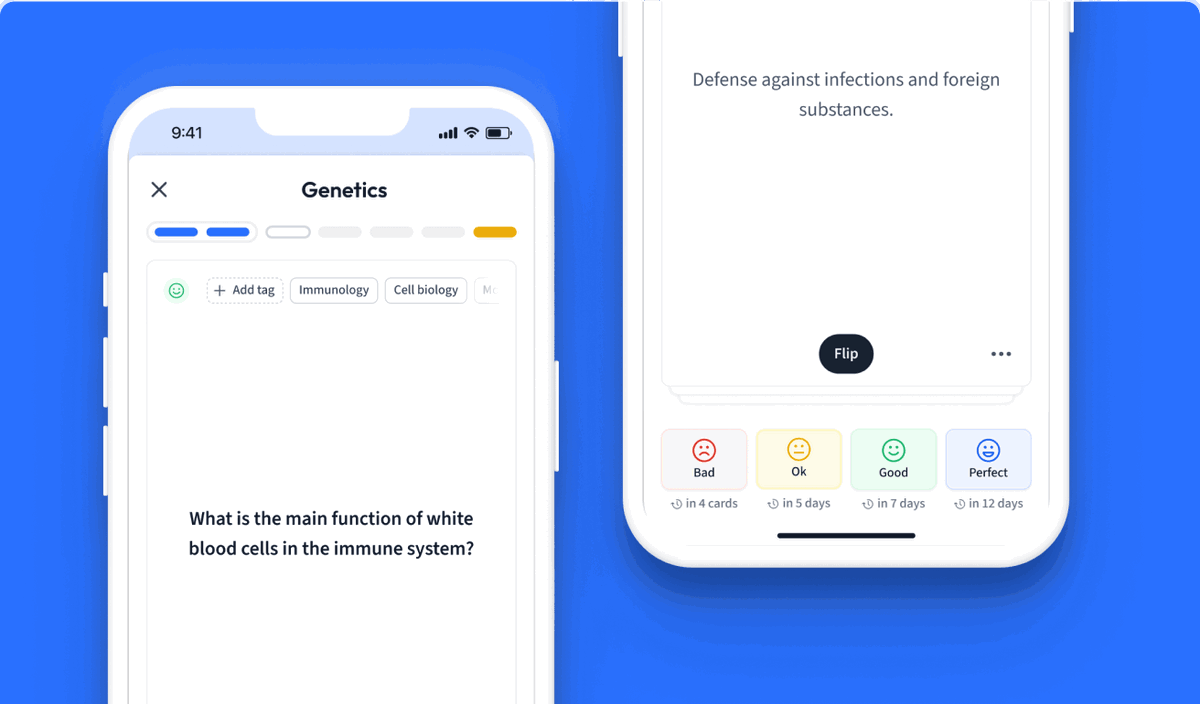
Learn with 26 Photoelectricity flashcards in the free StudySmarter app
We have 14,000 flashcards about Dynamic Landscapes.
Already have an account? Log in
Frequently Asked Questions about Photoelectricity
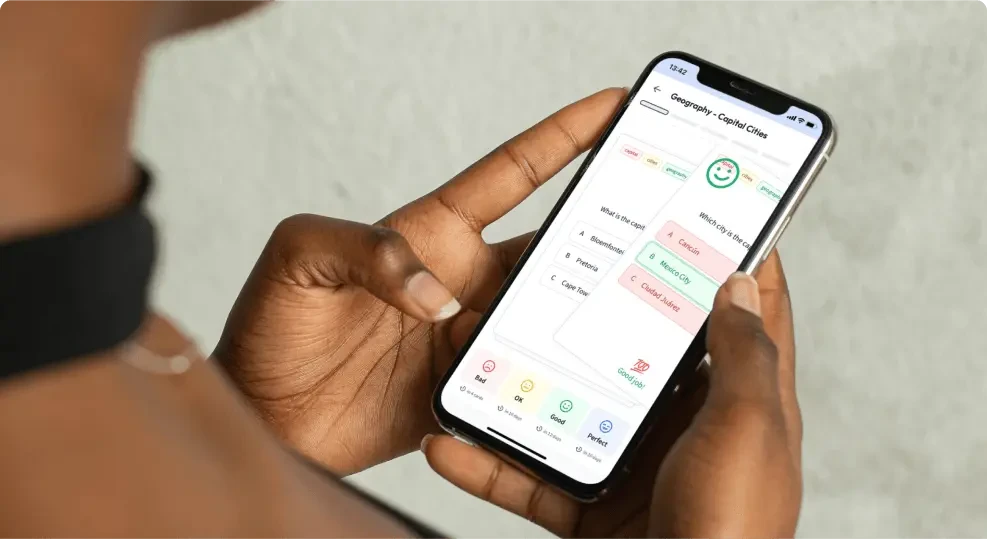
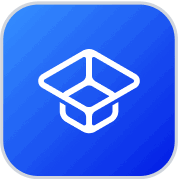
About StudySmarter
StudySmarter is a globally recognized educational technology company, offering a holistic learning platform designed for students of all ages and educational levels. Our platform provides learning support for a wide range of subjects, including STEM, Social Sciences, and Languages and also helps students to successfully master various tests and exams worldwide, such as GCSE, A Level, SAT, ACT, Abitur, and more. We offer an extensive library of learning materials, including interactive flashcards, comprehensive textbook solutions, and detailed explanations. The cutting-edge technology and tools we provide help students create their own learning materials. StudySmarter’s content is not only expert-verified but also regularly updated to ensure accuracy and relevance.
Learn more