Understanding Fluid Fundamentals
In the broadest sense, to develop your understanding of Fluid Fundamentals, it is crucial to start by defining what a fluid actually is, then delve deep into the essential properties that make a fluid distinctively distinguishable from other states of matter, such as solids.Defining Fluid: What is a Fluid?
As you embark on the journey of understanding Fluid Fundamentals, the very first stepping stone lies in defining what a fluid is. In technical terms, a fluid is any substance in a liquid or gaseous state that conforms to the shape of its container. This ability to flow and change shape under an applied force, distinguishes fluids from solids.A Fluid is a substance that deforms continuously under an applied shear stress. It includes liquids, gases and plasmas.
Comparison between Fluids and Solids
The key difference between solids and fluids lies in their response to shear stress.- While a solid can resist a shear stress, due to its rigid nature, a fluid cannot.
- Fluids, unlike solids, conform to the shape of the container they are in. This allows them to flow and occupy the entire volume of the container.
Unravelling the Properties of Fluid
As you journey deeper into the realm of Fluid Fundamentals, you will encounter several intrinsic properties of fluid which govern its behaviour under different conditions. These properties include, but are not limited to density, temperature, pressure, and viscosity. Each of these properties plays a pivotal role in how a fluid interacts with its environment and other substances.The Role of Temperature and Pressure in Fluid Properties
When it comes to fluid properties, both temperature and pressure play decisive roles. It's important to understand that these variables are interdependent, meaning a change in one can significantly affect the other. For example, if a gas is heated at constant volume, its pressure increases in accordance to Gay-Lussac’s law, represented as \[ P \propto T \].In reality, the equation is \(P = kT\), where \(P\) is the pressure, \(T\) is the temperature, and \(k\) is the proportionality constant. This equation suggests that, at constant volume, the pressure of a gas is directly proportional to its absolute temperature.
Viscosity: An Essential Fluid Property
Viscosity is another fundamental property of fluids. It is sometimes described as the 'stickiness' of a fluid and dictates how resistant the fluid is to deformation under shear stress. Think of how honey (a high viscosity fluid) flows slower than water (a low viscosity fluid). The formal definition of viscosity can be expressed as follows:Viscosity is the measure of a fluid's resistance to shear or flow, and it is commonly perceived as 'thickness'.
For instance, when you try to stir honey with a spoon, the resistance you face is due to its high viscosity. Whereas, stirring water, which is of a lower viscosity, is much easier.
Exploring the Domain of Fluid Kinematics
The vast landscape of Fluid Fundamentals extends further to a significant sub-discipline known as Fluid Kinematics. This crucial realm deals with the motion of fluids without considering the forces or pressures that cause the motion. Studying Fluid Kinematics helps engineers, scientists, and researchers better understand and predict fluid behaviours in various natural and man-made systems.Breaking Down Fluid Kinematics: Basics and Importance
Diving into the core concepts of Fluid Kinematics, it primarily involves studying and analysing the motion - or the lack thereof - of fluid particles. Commonsensically, the branch does not concern itself with the forces or pressures influencing these behaviours. Instead, it focuses on several key facets, including velocity fields, the acceleration of fluid particles, as well as the declaration of fluid flow - concepts that we will explore extensively in this section. By understanding the essentiality of Fluid Kinematics, you can gain the knowledge required for various applications in engineering and science. From designing sophisticated hydraulic systems to predicting weather patterns, the principles grounded in Fluid Kinematics play an instrumental role. An integral component of Fluid Kinematics is the analysis of fluid flow description. This concept melds three critical characteristics - streamlines, streaklines, and pathlines.
Description of Fluid Flow: Streamlines, Streaklines, and Pathlines
To comprehend fluid flow, you have to grasp the ideas of streamlines, streaklines, and pathlines. These are merely visualisations, graphical representations, or mathematical forms that can provide profound insights into fluid motion. A streamline refers to a curve that is tangential to the velocity vector of the flow. Mathematically, you can calculate the streamline using the following formula: \[ d\vec{r} = \vec{V} dt \] A streakline, on the other hand, is the path traced by particles of fluid that have previously passed through a common point. This is commonly visualised by introducing dye into a fluid and observing its trajectory. Compare that with a pathline, which represents the path travelled by a single fluid particle over a period of time. It's similar to tracing the journey of a single drop of dye in the fluid. By understanding these three descriptions of fluid flow, you can gain a more comprehensive understanding of how fluids behave under different conditions, allowing for accurate predictions in a variety of scenarios.How Fluid Kinematics Influences Engineering Fluid Mechanics
The principles derived from Fluid Kinematics are interconnected with the study of Engineering Fluid Mechanics - influencing it greatly. The manner in which fluids move and interact within different systems provides essential insights that allow engineers to design more efficient and functional fluid-dependent systems. Its applications span a vast range of sectors:- In the field of Civil Engineering, flow rates of water in rivers and streams are monitored using principles from Fluid Kinematics
- Mechanical Engineers utilise these principles in designing hydraulic systems, heating systems and coolant systems, to mention only a few
- Similarly, within the realm of Environmental Engineering, predicting the dispersion of pollutants in the atmosphere or bodies of water requires a deep understanding of Fluid Kinematics.
Fluid Fundamentals and Applications
Applications of Fluid Fundamentals are ubiquitous, shaping many aspects of our daily lives and the technologies we interact with. From the bubbles in your fizzy drinks to the flight of an aeroplane, the influence of fluid mechanics is far-reaching and profound.Real-World Applications of Fluid Fundamentals
Principles of Fluid Mechanics lay the foundation for numerous natural phenomena and technological inventions. These principles guide the design and operation of an array of diverse systems. Below are specific examples to illustrate just how integral Fluid Fundamentals are in the real world:- Aerodynamics: The science behind how air moves around objects is based on the principles of fluid mechanics. For example, the design of aeroplane wings (airfoils) is directly influenced by an understanding of fluid flow patterns, resistance, and lift generation. Aerodynamic design also impacts the fuel efficiency and speed of automotive vehicles.
- Hydrodynamics: Encompassing all matters related to water flow, hydrodynamics is crucial for understanding and predicting weather patterns, designing water treatment processes, and establishing effective irrigation systems.
- Heat Transfer: Fluids, especially gases and liquids, play a significant role in heat transfer applications. Principles of convection, one of the primary modes of heat transfer, hinge entirely on the movement of fluids.
By understanding the Fluid Fundamentals, we can optimise these systems for safety, efficiency, and cost-effectiveness. This knowledge serves as a foundation upon which real-world applications are designed and realised.
How Understanding Fluid Fundamentals Optimise Engineering Designs
Engineering designs across a multitude of disciplines hinge on the understanding of fluid movement and behaviours. Fluid Fundamentals not only guide the initial design process but also aid in troubleshooting, optimisation, and advancement of existing systems. Here's how:- Performance Improvement: Understanding the properties and behaviours of fluids allows for the optimisation of design, enhancing system performance and energy efficiency. For example, streamlining the shape of a vehicle can decrease air resistance, augment speed and improve fuel efficiency.
- Failure Prediction and Prevention: By understanding fluid behaviours, engineers can predict potential failures in a system. As such, preventative measures can be implemented, avoiding costly breakdowns and ensuring system safety.
- Emerging Technologies: Advances in areas like microfluidics and fluid power systems are made possible by leveraging a deep understanding of Fluid Fundamentals. These emerging technologies impact diverse fields, including healthcare and renewable energy.
Influence of Fluid Mechanics Fundamentals on Hydrodynamics and Aerodynamics
The sciences of Hydrodynamics and Aerodynamics are deeply rooted in the concepts of Fluid Mechanics. Both these disciplines study the motion of fluids - hydrodynamics is concerned with liquids (mainly water), while aerodynamics pertains to gases (primarily air). Hydrodynamics is vital in various sectors, including marine engineering, environmental engineering, and civil engineering. It assists in designing water sanitation systems, predicting coastal erosion, building efficient ships and underwater vehicles, and optimising renewable energy devices, such as hydroelectric turbines. As for aerodynamics, it holds critical roles in the design of aircrafts, automobiles, bridges and even windmills. For example, an aeroplane's wing shape - the airfoil - is designed to manipulate air pressures around the wing, generating lift. Similarly, for fuel-efficient and high-speed vehicles, designers seek to minimise air resistance, a concept known as drag. The study of flow patterns, pressure distribution, force calculations, viscous effects, and wave interactions all fall under these disciplines, and a solid understanding of Fluid Fundamentals is at the heart of it all. Whether it be to harness renewable energy or predict devastating storm surges, the influence of Fluid Mechanics Fundamentals on Hydrodynamics and Aerodynamics cannot be overstated.Comprehensive Study of Fluid Mechanics Fundamentals
The comprehensive study of Fluid Mechanics Fundamentals is an essential pursuit within several engineering disciplines. From civil engineering, where it is used to model fluid movement in transport and infrastructure, to mechanical and aerospace engineering, which requires understanding of fluid behaviour to design efficient systems and vehicles, the scope is wide and impactful. Bearing such overarching importance, it's vital to delve into the intricacies of Fluid Mechanics' key principles and fundamental equations.Relevance of Fluid Mechanics Fundamentals in Engineering
Indeed, Fluid Mechanics Fundamentals permeates far and wide into various engineering domains, playing a significant role in the formulation of effective and efficient solutions. Being an imperative part of an engineer's toolkit, these principles facilitate understanding the behaviour and interaction of fluids within a multitude of circumstances. For instance, civil engineers heavily rely on these principles to solve issues stretched across water treatment systems, dam designs, and canal systems. Similarly, in the aviation industry, aircraft designs are optimised using fluid principles to improve aerodynamics, thus enhancing fuel efficiency and performance. Meanwhile, mechanical engineers employ the principles during the creation of sophisticated fluid machinery, including pumps and compressors. Furthermore, environmental engineers utilise the principles for modelling the dispersion of pollutants in various media—air, water, and even soil. In chemical engineering, fluid mechanics is employed to design and optimise reactors and distillation columns, where fluid flow is a central element. Process efficiency, safety, and environmental compliance—all depends on skilled manipulation and understanding of fluid behaviour.- Civil Engineers utilise Fluid Mechanics Fundamentals to design efficient water supply and treatment systems.
- Mechanical Engineers use these principles to design effective coolant systems, pumps, and compressors.
- Chemical Engineers rely on these concepts to design reactors and distillation columns.
Equations Governing Fluid Mechanics Fundamentals
The concise depiction of fluid principles can be spotted within the womb of specific fundamental equations critical to fluid mechanics. These equations include the Continuity Equation, the Navier-Stokes Equation, and the Bernoulli's Equation, each serving unique purposes and revealing different facets of fluid behaviour. The Continuity Equation derives from the law of conservation of mass. In the context of a fluid flowing through a pipe, it implies that the mass entering the system equals the mass leaving the system. Mathematically, it can be stated as: \[ \frac{\partial \rho}{ \partial t} + \nabla \cdot (\rho \vec{V}) = 0 \] Here, \( \rho \) denotes fluid density, \( t \) represents time, and \( \vec{V} \) is the fluid velocity vector. Meanwhile, the Navier-Stokes Equation is substantially more complex. It represents Newton's second law of motion for fluids. In simpler words, it talks about the balance of forces acting on a fluid element. This equation considers factors like pressure, viscosity, and external forces, such as gravity. \[ \rho \frac{D\vec{V}}{Dt} = -\nabla P + \mu \nabla^2 \vec{V} + \rho \vec{g} \] In the above equation, \( P \) stands for pressure, \( \mu \) represents viscosity, and \( \vec{g} \) is the gravitational force vector. Lastly, Bernoulli's Equation deals with the conservation of energy principle for flowing fluids, considering kinetic energy, potential energy, and internal energy due to pressure. Abstracted to a simple and idealised form, the equation is represented as: \[ P + \frac{1}{2} \rho V^2 + \rho gh = constant \] Here, \( V \) represents the fluid velocity, \( h \) is the height or elevation, and \( g \) corresponds to the acceleration due to gravity.Role of Assumptions in Fluid Mechanics Fundamentals
In practice, fluid behaviour can get quite perplexing with myriad factors at play. Therefore, to forge practical applications and manageable analyses, certain assumptions come in handy. They simplify the complex real-world problems into manageable mathematical models, paving the way for practical solutions. However, it’s crucial to note that assumptions should be made judiciously as erroneous assumptions can lead to inaccurate results. Some of the commonly made assumptions in fluid mechanics include incompressibility of fluid, steady flow and inviscid fluid. For instance, the assumption of incompressibility—considering the fluid density to be constant—is convenient and reasonably accurate for liquids and low-speed gas flows, as their densities do not change significantly under normal conditions. Steady flow assumes that fluid properties at any point in the system don't change with time. This is a reasonable presumption for systems in a stable operation phase and provides greatly simplified calculations. Inviscid fluid assumption negates the influence of viscosity. This is feasible in situations where viscous forces are negligible compared to other forces—like high-speed gas flows. While these assumptions greatly simplify the calculations, they also abstract away from the realism of fluid behaviour, essentially serving as an approximation rather than an exact depiction of fluid flow.Diving into Fundamentals of Fluid Dynamics
Fluid Dynamics, an essential subset of Fluid Mechanics, revolves around the science of fluid (liquid and gas) in motion. This fundamental concept helps in analysing and predicting fluid flow patterns, making it a pillar of various engineering branches, from aeronautics to civil engineering. Offering comprehensive insights into fluid behaviour under various conditions, fluid dynamics facilitates the design and development of various engineering systems and structures.Introduction to the Concept of Fluid Dynamics
Fluid Dynamics is an intriguing branch of physics focussed on studying the forces and movement of fluids. It encapsulates smaller domains such as hydrodynamics and aerodynamics, which specifically denote the study of liquid and gas flows, respectively. An understanding of fluid dynamics is integral for engineers and scientists seeking solutions to problems involving the motion of fluids. For instance, aerospace engineers leverage fluid dynamics to devise streamlined aircraft shapes that minimise drag and boost fuel efficiency. Similarly, civil engineers rely on fluid dynamics to design efficient water supply systems by predicting and controlling flow rates. Additionally, environmental scientists apply fluid dynamics to understand and mitigate issues like pollution dispersion and water contamination.
Fluid Dynamics: A branch of physics that studies the forces and flows of fluids (liquids and gases).
Equation of Motion in Fluid Dynamics: Momentum Equation
In fluid dynamics, the equation of motion or momentum equation is a crucial mathematical model reflecting Newton's second law in fluids. This equation is critical in describing how the velocity of a fluid changes in response to external forces. The momentum equation can be depicted as: \[ \frac{{D(\rho \vec{v})}}{{Dt}} = -\nabla P + \nabla \cdot [ \rho (\vec{v} \otimes \vec{v}) ] + \vec{F} \] In this equation, \( \rho \) signifies fluid density, \( \vec{v} \) represents the velocity vector, and \( P \) stands for pressure. Additionally, \( \vec{F} \) accounts for the volume forces, with \( \vec{g} \) (gravity) being most common. This equation states that the rate of change of momentum of a fluid parcel is equal to the sum of the pressure and viscous forces acting on it. The equation unveils significant insight about pressure distribution and flow patterns in fluid systems.Analysing the Application of Fluid Dynamics in Engineering
In the realm of engineering, the applications of fluid dynamics span far and wide, seamlessly merging into multiple fields. In aerospace engineering, fluid dynamics helps decipher the intricacies of air flow over aircraft wings to maximise aerodynamic lift and minimise drag. Understanding fluid dynamics facilitates the development of efficient aircraft designs that resonate with enhanced fuel efficiency and improved flight performance. The repercussions of fluid dynamics are also prominent in designing space shuttles and rockets, wherein it aids in interpreting the effects of atmospheric friction on the vehicle’s shape and material.The science of Fluid Dynamics plays a decisive role in shaping up the wing designs of aircraft, enhancing the overall efficiency and reducing the fuel consumption.
Aerospace Engineering | Improving aircraft designs, minimising drag, interpreting effects of atmospheric friction. |
Civil Engineering | Designing water treatment systems, dams, canals, and sizing distribution pipes. |
Mechanical Engineering | Designing fluid machinery; Enhancing heat transfer in thermal management systems. |
Computer Graphics | Creating visually stunning simulations of phenomena like smoke, fire, water. |
Fluid Fundamentals - Key takeaways
- Fluid Kinematics involves studying the motion of fluid particles without considering the forces or pressures involved. It includes the study of velocity fields, the acceleration of fluid particles, and the analysis of fluid flow.
- Three key concepts used to describe fluid flow are streamlines, streaklines, and pathlines; streamline refers to a curve tangential to the velocity vector of the flow, streakline is the path traced by particles that have previously passed through a common point, and pathline represents the path travelled by a single fluid particle over time.
- Fluid Kinematics principles have various applications in engineering and science, including Civil Engineering and Mechanical Engineering. It helps in designing hydraulic systems, predicting weather patterns, and analyzing fluid-dependent systems.
- Fluid Fundamentals and its applications are present in our daily lives and influence several technologies, including aerodynamics (science of how air moves), hydrodynamics (related to water flow), and heat transfer applications.
- Fundamentals of Fluid Dynamics, a subset of Fluid Mechanics, is key for analysing and predicting fluid flow patterns and influences various fields like aeronautics and civil engineering.
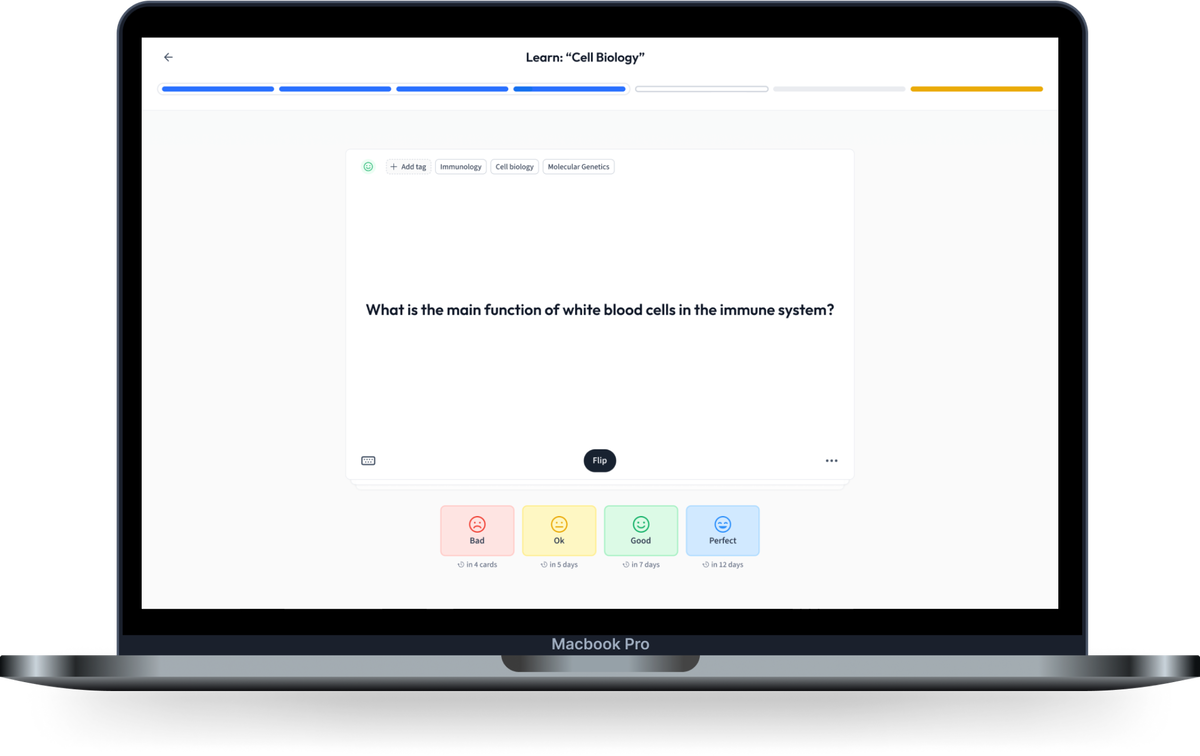
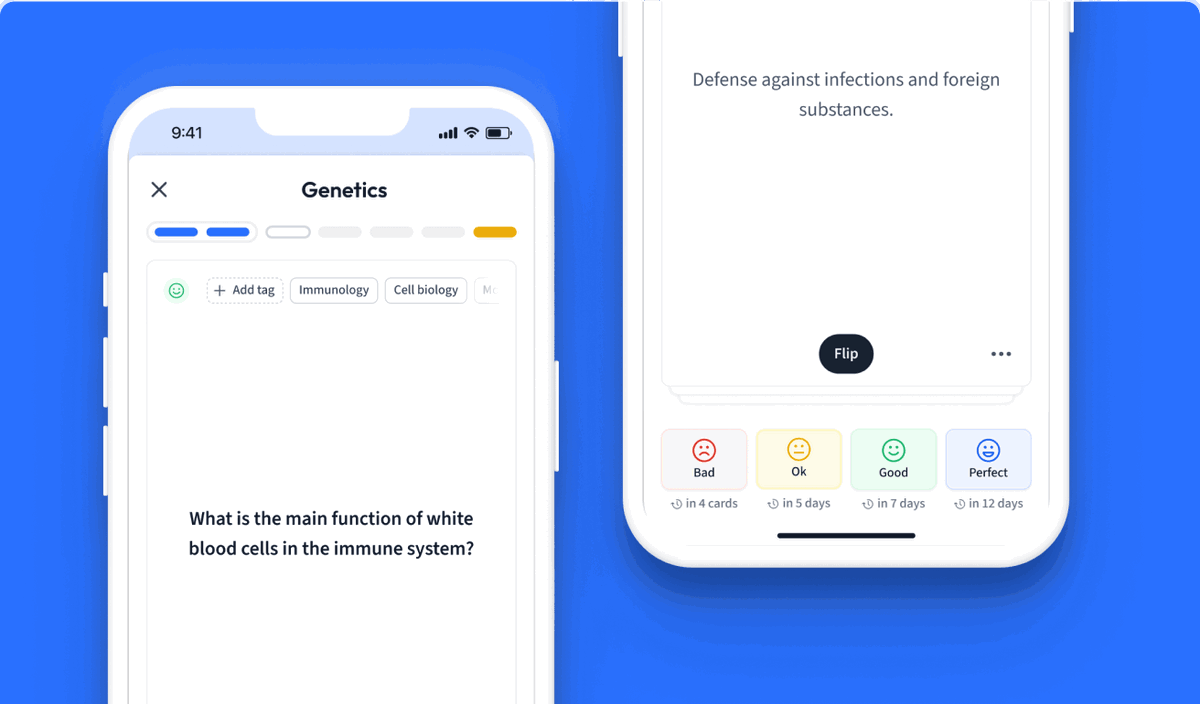
Learn with 740 Fluid Fundamentals flashcards in the free StudySmarter app
We have 14,000 flashcards about Dynamic Landscapes.
Already have an account? Log in
Frequently Asked Questions about Fluid Fundamentals
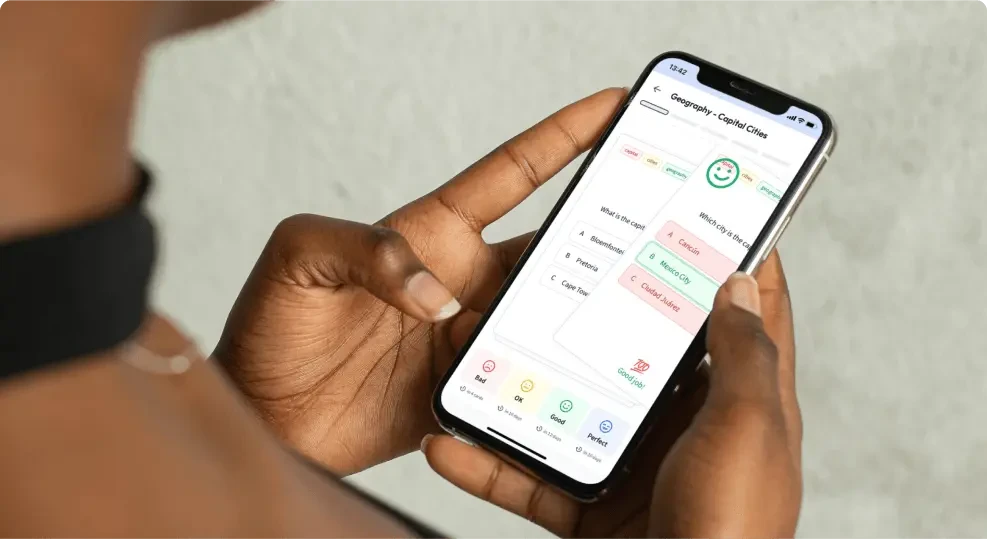
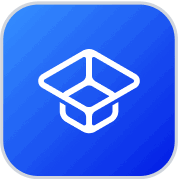
About StudySmarter
StudySmarter is a globally recognized educational technology company, offering a holistic learning platform designed for students of all ages and educational levels. Our platform provides learning support for a wide range of subjects, including STEM, Social Sciences, and Languages and also helps students to successfully master various tests and exams worldwide, such as GCSE, A Level, SAT, ACT, Abitur, and more. We offer an extensive library of learning materials, including interactive flashcards, comprehensive textbook solutions, and detailed explanations. The cutting-edge technology and tools we provide help students create their own learning materials. StudySmarter’s content is not only expert-verified but also regularly updated to ensure accuracy and relevance.
Learn more