Understanding Shoaling in Engineering Fluid Mechanics
Shoaling is a term you'll come across often in engineering fluid mechanics, particularly in fields like civil and coastal engineering. It describes the transformational process that waveforms undergo when transitioning from deep water to shallower areas, usually near the shoreline. This process is incredibly important in engineering because it influences several aspects, such as the design of coastal structures, prediction of erosion patterns, and development of flood prevention strategies.
Defining the Concept of Engineering Shoaling
In engineering fluid mechanics, shoaling refers to the way waves change their characteristics as they move from deep to shallow water. It's interesting to note that these changes happen in two distinct stages, deep-water and shallow-water shoaling.
Deep-water shoaling occurs as waves transition from the ocean depths and reach waters often less than half their original wavelength. The waves' velocity decreases and their height increases, which leads to a change in wave shape and period.
Subsequently, in shallow-water shoaling, the wave energy is dispersed over increasingly smaller water volumes. This energy concentration results in significant wave height increases.
Core Mathematics Involved in Shoaling Technique
Shoaling involves intricate aspects of physics and mathematics, which are fundamental to understanding wave behavior in fluid mechanics. These mathematical principles include wave propagation, wave dispersion, and wave transformation, each playing a key role in shoaling.
Let's start with wave propagation. In open waters, waves propagate according to the deep water dispersion relation:
\[h = \frac{g} {2\pi} \times \frac{T^2} {tanh\left(\frac{2\pi h}{T} \right)}\]The height of the wave "h" depends on the gravitational acceleration "g", the wave period "T", and the water depth "h".
The dispersion relation takes into account several factors including the wavelength, water depth, and wave period. It is key to predicting wave propagation in deep waters and helps engineers estimate potential wave behavior as they approach the shoreline.
Real Life Shoaling Examples in Civil Engineering
In the realm of civil engineering, the concept of shoaling is critical in designing and implementing various coastal structures and measures. From levee systems to breakwaters and harbor structures, understanding the effects of shoaling helps in predicting the impact of waves on structures and the surrounding environment.
Consider the construction of a harbor. The design must account for potential wave heights to ensure the structures are capable of withstanding the forces. Engineers use calculations based on shoaling theory to predict the wave behavior, determining how waves will change as they move from deep to shallow water, and structuring the harbor accordingly. This aspect of design is essential in maintaining the durability and safety of maritime structures.
Shoaling Causes and Their Effects in Civil Engineering
While the general principle of shoaling is understood in the context of fluid mechanics, particularly the way wave characteristics transform as they transition from deep to shallower water, it's equally important to discuss the potential causes of shoaling and their subsequent effects on civil engineering projects. These causes aren't always straightforward, often involving a complex interplay of geophysical, meteorological, and human-induced factors.
Possible Factors Causing Shoaling in Engineering
There are numerous potential influencers of shoaling. These include natural factors like tidal activity, weather conditions, and landforms, as well as anthropogenic activities such as development projects, pollution, and water traffic. It's essential to understand each of these in order to predict, mitigate, or adapt to any changes in wave behaviour caused by shoaling.
- Tidal Activity: Both lunar and solar tides influence wave patterns and control the water depth, altering the forces exerted by waves on the shoreline.
- Weather Conditions: Weather conditions such as storms, winds, and pressure systems can dramatically increase wave heights and cause heightened shoaling effects.
- Landforms: The topography of the sea floor significantly impacts the direction, speed, and shape of waves. This includes the slope of the sea bed, underwater formations, and coastal outline.
- Development Projects: Infrastructure projects, dredging, cliff stabilisation, and other such activities can drastically alter sea bed profiles, thus modifying wave patterns and causing shoaling.
- Pollution: Activities that contribute to water contamination may influence water density, which in turn affects wave propagation and behaviours.
- Water Traffic: Large-scale water traffic, including container ships and ferries, may temporarily change water levels and affect long-term wave patterns leading to shoaling.
Analysing the Effects of Shoaling in Civil Engineering Projects
An understanding of shoaling is integral to the successful completion of civil engineering projects, particularly those by the coastline or offshore. From simple dock construction to complex offshore wind farms, engineers need to account for how shoaling can impact wave behaviour and how this, in turn, affects the structures they are designing.
Shoaling can lead to various outcomes:
- Increased Wave Heights: As waves move from deeper to shallower water, their heights increase significantly. This can impose greater force on the structures, which must be designed to withstand this additional stress.
- Erosion and Deposition: Shoaling can cause increased erosion to coasts and lead to deposition in some areas, altering local landscapes and potentially threatening coastal structures and infrastructure.
- Increased Risk of Flooding: When shoaling occurs near settlements, like towns and cities, and there are no adequately designed coastal defences, it could result in devastating floods.
Case Studies: The Destructive Impact of Shoaling
There have been several notable instances in the past where shoaling has directly led to significant damage to engineering structures or posed challenges for existing infrastructure. Below are a few examples:
The Port of Long Beach, USA: The port is considered one of the world's busiest seaports. Over the years, heavy-duty traffic combined with natural factors has led to extreme shoaling in the port's main channel, necessitating regular and costly dredging operations.
Venice, Italy: The famed city of canals struggles with increased shoaling owing to heavy boat traffic, tidal motion, and local atmospheric conditions. This has led to irregular water levels in the canals, affecting not only the existing buildings and infrastructure but also the city's future building projects.
These examples underline the grave impact shoaling can have if not accurately predicted and properly managed as part of an engineering project's planning and implementation stages.
The Interconnection Between Shoaling and Fluid Dynamics
Engineering fluid dynamics and the concept of shoaling - alterations in wave characteristics moving from deep to shallow water - are deeply interconnected. This relationship forms a pivotal aspect of fluid dynamics study and is instrumental in efficiently managing coastal and offshore engineering projects. A thorough understanding of how fluid dynamics and shoaling intersect can offer insightful tools to engineers tackling fluid mechanistic challenges.
Exploring Shoaling in the Context of Fluid Dynamics
For a comprehensive understanding of how shoaling is part of fluid dynamics, let's dig deeper into the principles of fluid dynamics themselves. Fluid dynamics explores the influence of forces on fluid motion. It's an offshoot of fluid mechanics, the branch of physics that studies fluids (gases and liquids) at rest and in motion. An array of mathematical models and physical laws describe these interactions. Two essentials to highlight are the Continuity Equation and Navier-Stokes Equation.
The Continuity Equation expresses the principle of conservation of mass for fluid systems. It states for any volume of fluid moving through a system, the mass flow rate into the volume is equal to the mass flow rate out of the volume plus the rate of change of the mass within the volume. The simplified equation is: \[ \frac{\partial \rho}{\partial t} + \nabla \cdot (\rho u)=0 \] where 'ρ' is the fluid density, 't' is time, 'u' is the fluid velocity, and '∇' is the divergence operator.
The Navier-Stokes Equations, a set of differential equations, describe fluid flow by combining the fundamental laws of conservation of momentum and Newton's second law. It is written as: \[ \rho \left( \frac{\partial \mathbf{u}}{\partial t} + \mathbf{u} v \mathbf{u} \right) = -\nabla p + \nabla \cdot \mathbf{T} + \mathbf{f} \] where 'ρ' is the fluid density, 'u' is the fluid velocity, 't' is time, 'p' is the pressure, '∇' is the gradient operator, 'T' is the stress tensor and 'f' is the body forces.
Interpreting waves through the prism of fluid dynamics, and particularly applying the mentioned equations, help engineers in predicting, modelling, and managing the shoaling process. Waves travelling toward a shoreline, going through deep-water to shallow-water shoaling, can be observed as fluid particles transitioning from circular to elliptical to linear paths, while their heights and trajectories change due to the influence of factors such as water depth, wave period, and wavelength.
How Fluid Dynamics Factors Influence Shoaling Phenomenon
Several fluid dynamics principles play a crucial role in the shoaling phenomenon. A plethora of variables are at play when it comes to the modifications waves go through as they approach shallow water. The following factors of fluid mechanics are particularly significant for analysing how shoaling occurs:
- Wave Speed and Water Depth: Waves slow down as the water gets shallower, leading to wavefront compression and an increase in wave height.
- Wave Dispersion: Dispersion, or the separation of waves by speed and direction, is affected by water depth and shoreline contour - thus affecting how wave energy propagates and is distributed.
- Wave Refraction: This is the bending of waves as they approach a shoreline at an angle and is significantly affected by changes in water depth - thereby playing a key role in shoaling.
- Wave Diffraction: The spreading out of wave energy when it encounters an object or gap. Through diffraction, wave energy can be guided into bays and harbours, leading to shoaling.
By analysing these variables in the context of fluid dynamics, engineers can predict and prepare for the consequences of shoaling, enabling them to safeguard structures, mitigate erosion and flooding risks, and determine the best sites for offshore projects.
Practical Examples: Shoaling and Fluid Dynamics in Engineering Mechanics
Engineers often rely on numerical models and simulations to address how waves may impact a structure or an area. These predictive models incorporate fluid dynamics equations and principles to predict how shoaling might occur and what its influence may be on intended projects. Let's delve into some practical applications:
Offshore Wind Farms: When planning where to place offshore wind turbines, engineers must consider how waves will interact with the structures. Attention must be given to shoaling patterns that could lead to larger-than-anticipated wave forces on the turbine towers, affecting their stability and longevity. Using fluid dynamics to predict shoaling behaviour can aid in safe and effective positioning of turbines.
Marinas and Harbours: Marinas and harbours need to balance the need for access with protection from wave action. Shoaling patterns can lead to sediment build-up, requiring frequent and costly dredging operations. Fluid mechanics principles and models help in understanding these patterns, allowing engineers to design structures optimally to minimise shoaling and associated problems.
These real-world instances underpin the relevance of fluid dynamics knowledge in predicting and managing shoaling and demonstrate its considerable impact on engineering project planning and implementation.
Different Types of Shoaling in Engineering
Shoaling, the tendency of waves to change characteristics as they move from deep to shallow water, is a critical phenomenon in engineering. There are notably different types of shoaling with varying characteristics that occur under diverse conditions. These types have different implications and are considered in planning and executing various civil engineering projects.
Classifying Various Shoaling Types in Civil Engineering
In Civil Engineering, the types of shoaling are primarily categorised by noting wave behaviour as they approach the shorelines. Distinctive classifications that emerge include Normal Wave Shoaling, Spilling Breakers, Plunging Breakers, and Surging Breakers.
- Normal Wave Shoaling: In normal wave shoaling, the waves exhibit increased height and reduced speed as they encounter shallow water, but they do not break. The wave length decreases and the crest of the wave becomes steeper.
- Spilling Breakers: Spilling breakers occur when waves break over a considerable distance, spilling water over the crest and down the front of the wave. This process often takes place over gently sloping or flat sea beds, leading to a gradual energy release.
- Plunging Breakers: In contrast, plunging breakers, which are typically hollow waves breaking over steeper sea beds, exhibit a fast and abrupt energy release. The crest of the wave curls over and plunges downward, causing a lot of spray and foam.
- Surging Breakers: These are observed when waves break almost directly onto the beach or structure. They usually occur on very steep slopes, where the wave energy is rapidly dissipated and the wave may surge up the slope.
Each of these types of shoaling is observed under differing conditions of depth, slope, and wave pattern. Understanding the formation and behaviour of these types allows engineers to anticipate and manage their effects on coastal structures and activities.
In-depth Analysis of Each Type of Shoaling
Considering the significant roles these differing shoaling types play in coastal and offshore planning, understanding their mechanics and resultant repercussions becomes imperative.
Normal Wave Shoaling: As waves propagate from deep to shallow water, their speed decreases while their amplitude (or height) increases. This phenomenon is a result of the energy conservation principle, modelled in fluid dynamics by the equation \[\sqrt {\frac {g}{k \cdot \tanh (k \cdot d)}} \] where 'g' is the acceleration due to gravity, 'k' is the wave number, 'd' is the water depth. 'tanh' designates the hyperbolic tangent function. Normal Wave Shoaling can result in changes in wave direction causing refraction and potential hazards to coastal structures.
Spilling Breakers: Commonly seen on flat, sandy beaches with a gentle slope, these breakers occur over a lengthier distance, leading to a slow surge of wave over time. This prolonged energy dispersal can result in extensive alongshore currents and cause significant coastal erosion issues.
Plunging Breakers: Occurring over a steeper slope, this type of breaker sees the top part of the wave overtake the bottom part and curl forward, plunging downwards. These high-energy breakers can cause considerable beach erosion and damage to structures but can also contribute to the creation of sandbars.
Surging Breakers: These breakers, moving rapidly up and down a steep shoreline slope without rolling over, can suddenly release energy onto the beach or coastal structures causing significant damage. Surging breakers can peel off shorelines, removing beach material and potentially undermining structures.
Types of Shoaling: Field Examples and Analysis
Real-world instances offer a concrete perspective of how the described classifications play out and impact our coastal areas. An array of examples illustrates these implications:
Normal Wave Shoaling: In offshore wind farm planning, careful attention to normal wave shoaling behaviour helps optimise the placement of turbines, ensuring they won't be adversely impacted by the increased wave heights and resultant loads.
Spilling Breakers: An instance of such shorelines could be the Brighton Beach in the UK, known for its flat-to-moderately sloping sandy beach where spilling breakers are common. These can lead to the requirement for regular beach nourishment and coastal protection measures.
Plunging Breakers: Surfing destinations like Bournemouth Beach Pier in the UK offer classic examples of plunging breakers. Plunging breakers create attractive environments for recreational activities like surfing, but the rapid energy release can cause issues with coastal erosion.
Surging Breakers: Rocky coastlines with steep slopes, like those found in parts of Cornwall in the UK, frequently experience surging breakers. These can lead to intense coastal erosion and are a significant consideration in coastal defence design and cliff stability assessments.
The practical understanding and examples of these types of shoaling offer engineers essential insights that influence design decisions, project implementation, and environment management strategies.
Techniques to Counteract The Effects of Shoaling
Mitigating the impact of shoaling is essential for the sustainability of coastal projects, and two main strategies can be adopted - proactive and reactive. Proactive measures are implemented to prevent excessive shoaling effects, while reactive techniques are employed to manage shoaling after its occurrence.
Proactive Measures to Mitigate Shoaling in Civil Engineering
Proactive measures include strategies that are implemented during the design and construction phases of a project to inhibit shoaling effects. These measures range from the structure's positioning to specifics of its design and construction.
Structural Orientation: Often, the first consideration in avoid shoaling impacts is the strategic placement and orientation of structures relative to prevailing wave patterns. This reduces the wave energy reaching the structures, thereby reducing the impact of shoaling. For example, structures can be oriented perpendicular to the wave direction to reduce wave loads.
Coastal Defence Structures: These are designed to reduce the power of waves reaching the shore, effectively reducing foreshore erosion, which is a common repercussion of shoaling. Examples of such structures include seawalls, breakwaters, and revetments.
Soft Engineering Techniques: These methods incorporate the use of natural processes to mitigate coastal erosion and flooding, subsequently reducing shoaling impacts. For instance, beach nourishment (addition of large amounts of sand or sediment to the beach) and dune stabilisation are common soft engineering techniques.
Contouring: This involves modifying the seabed's slope to manage wave transformation and reduce shoaling effects. This is commonly used in conjunction with beach nourishment and may involve dredging or depositing material.
Engineering Solutions to Prevent Adverse Effects of Shoaling
In addition to the proactive measures, reactive approaches are often required when shoaling effects have already occurred. These strategies are implemented to manage and reverse the effects of shoaling on existing structures and beach fronts.
Dredging: Often used as a reactive measure to restore water depth in channels and harbours affected by shoaling and sedimentation, dredging involves the removal of accumulated sediment from the seafloor.
Coastal Armouring: To protect against coastal erosion caused by shoaling, structures such as seawalls, bulkheads, and revetments are constructed. This essentially replaces the natural beach system and mitigates shoaling impacts.
Sediment Traps: These are designed to capture sediment moving along the seafloor to reduce deposition in critical areas (like navigation channels) influenced by shoaling.
Adaptive Structures: Certain structures can be designed to adapt to varying water depths and wave conditions. For instance, floating breakwaters rise and fall with the water level, maintaining their effectiveness even with shoaling-induced changes in water depths.
Real World Examples of Techniques Managing Shoaling Issues
Examples from around the world clearly illustrate the implementation of the mitigating techniques. They provide insight into how these strategies play out in real-world scenarios and the challenges and solutions associated with them.
Netherlands: With 2/3 of the country vulnerable to flooding, the Dutch have a long history of implementing both proactive and reactive measures to manage shoaling effects. This includes a wide range of coastal defences, from extensive seawalls and dikes to innovative soft engineering techniques like the 'Sand Motor' project, which uses natural wave action to disperse sand along the coast, replenishing beaches and combating coastal erosion.
Port of Long Beach, USA: This port has effectively employed dredging and sediment traps to manage sedimentation and shoaling in its navigation channels. They have also implemented a programme to reuse the dredged material beneficially, contributing to beach nourishment and wetland restoration projects.
Gold Coast, Australia: Known for its vibrant beaches, the Gold Coast has successfully employed a range of techniques to manage shoaling impacts, including beach nourishment, a network of groynes to manage longshore drift and an offshore artificial reef to dissipate incoming wave energy and reduce shoreline erosion.
These examples show a broad range of techniques applied across the world to adequately manage shoaling issues. They underscore the importance of considering local conditions, available resources, and long-term sustainability when selecting and implementing these solutions.
Shoaling - Key takeaways
- Shoaling: The phenomenon where wave characteristics change as they move from deep to shallow water. It plays a significant role in engineering, especially in coastal or offshore projects.
- Effect of shoaling: Leads to increased wave heights, erosion, deposition and an increased risk of flooding. It can have major implications on infrastructure and coastal settlements.
- Shoaling and fluid dynamics: The relationship between these two concepts is pivotal to managing coastal and offshore engineering projects. Practical understanding can help predict and manage the shoaling process, involving factors like water depth, wave period, and wavelength.
- Types of Shoaling: Varied types of shoaling occur under diverse conditions, including Normal Wave Shoaling, Spilling Breakers, Plunging Breakers, and Surging Breakers. Each of these has different implications for coastal structures and activities.
- Shoaling examples in engineering: For instance, shoaling patterns impact the placement of offshore wind turbines and the design of harbours and marinas. Real-world instances underline the importance of predicting and managing shoaling in such projects.
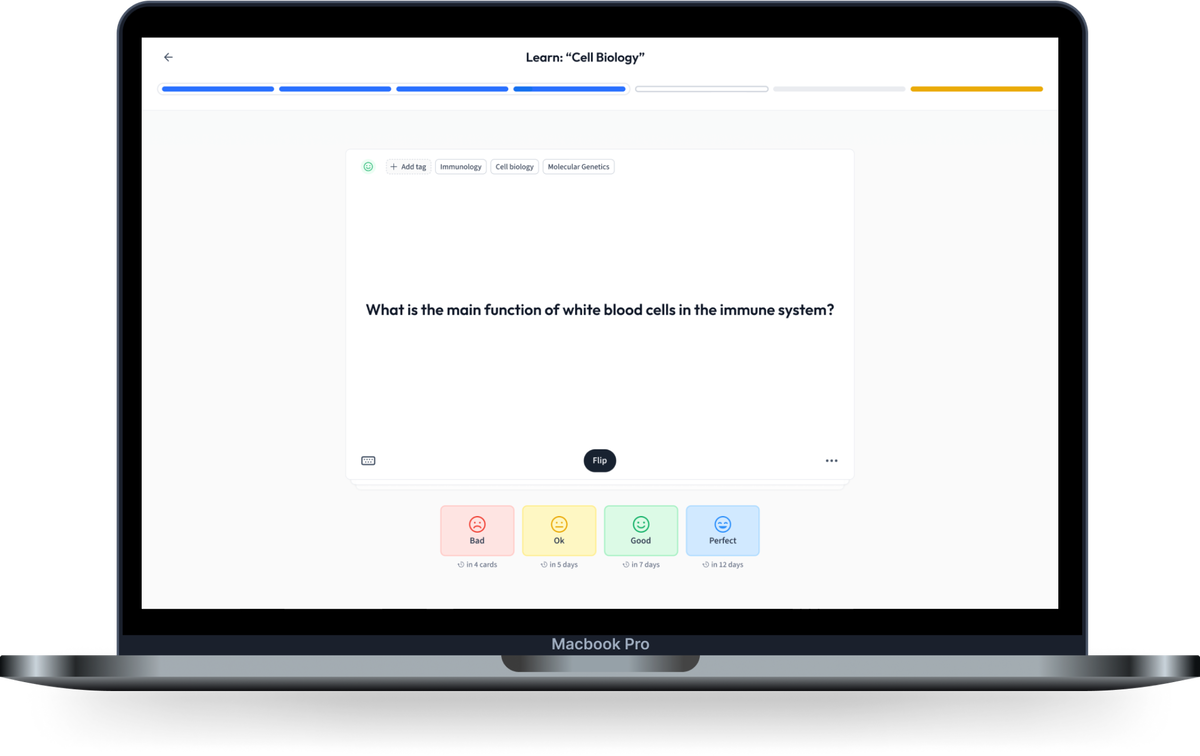
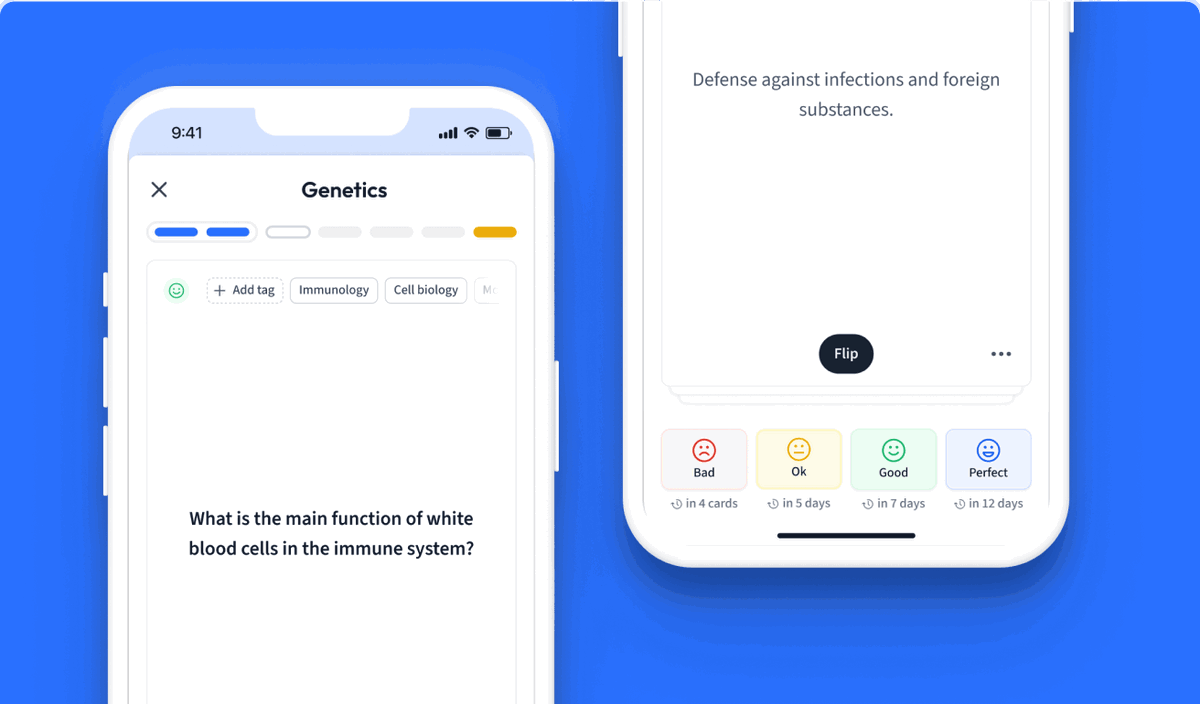
Learn with 15 Shoaling flashcards in the free StudySmarter app
We have 14,000 flashcards about Dynamic Landscapes.
Already have an account? Log in
Frequently Asked Questions about Shoaling
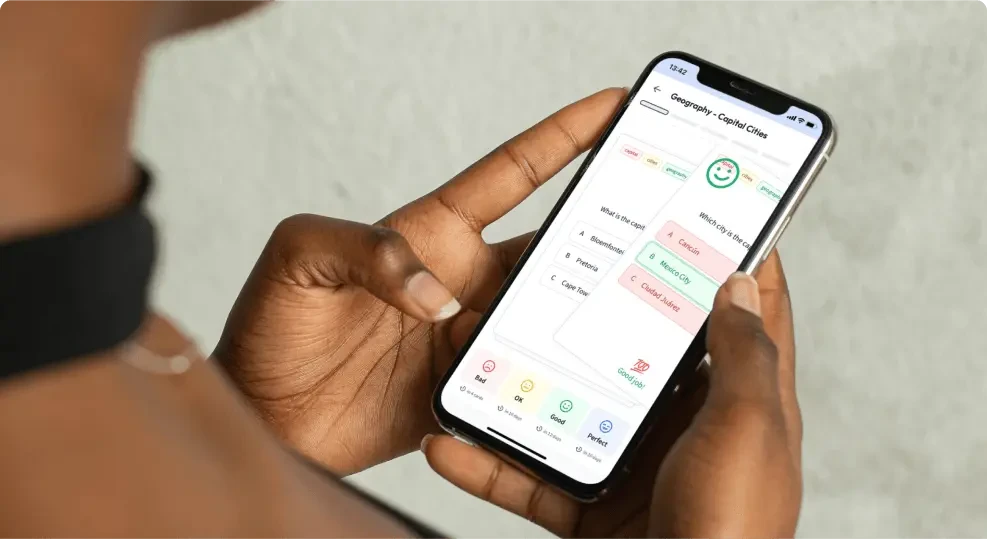
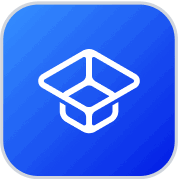
About StudySmarter
StudySmarter is a globally recognized educational technology company, offering a holistic learning platform designed for students of all ages and educational levels. Our platform provides learning support for a wide range of subjects, including STEM, Social Sciences, and Languages and also helps students to successfully master various tests and exams worldwide, such as GCSE, A Level, SAT, ACT, Abitur, and more. We offer an extensive library of learning materials, including interactive flashcards, comprehensive textbook solutions, and detailed explanations. The cutting-edge technology and tools we provide help students create their own learning materials. StudySmarter’s content is not only expert-verified but also regularly updated to ensure accuracy and relevance.
Learn more