Understanding Closed System Thermodynamics
In the vastness of engineering studies, you may come across the principle of Closed System Thermodynamics quite often. This integral concept of thermodynamics revolves around systems in which no mass may enter or leave.
The Meaning of Closed Systems in Thermodynamics
Technically, a closed system refers to a type of thermodynamic system where energy can be transferred in or out, but not mass. This is one of the most fundamental concepts encountered in thermodynamic studies.
A closed system allows the transfer of energy (heat and work), but it prohibits the transfer of mass. The boundary that encases this system may change its shape or volume, but always remains impermeable to matter.
The key equation that governs the behaviour of closed thermodynamics systems is the First Law of Thermodynamics, mathematically represented by:
\[ \Delta U = Q - W \]
Where:
\(\Delta U\) | is the change in internal energy, |
\(Q\) | is the heat added to the system, and |
\(W\) | is the work done by the system. |
Understanding closed system thermodynamics is pivotal in conceptualising conservation of energy and matter principles which happen to be the foundation stones of all physical sciences.
The Role of Closed Systems in Thermodynamics
Closed systems play a pivotal role in thermodynamics. Such systems help to explain the energy balance of a system and can be utilised to examine a wide range of phenomena.
Examples of Closed Systems in Thermodynamics
There are several practical instances of closed systems in the realm of thermodynamics. A few examples include water in a boiler or the refrigerant in an air conditioning system.
- A gas in a piston-cylinder assembly
- A steam in a steam generator
- Refrigerant in a refrigerator coil
- A mass of fluid being transported by a pump or fan
Everyday Examples of Closed Systems in Thermodynamics
You are in continual interaction with closed systems in everyday life. For instance, a pressured aerosol spray can is an excellent example of a closed system. The can's content doesn't change by mass, but when sprayed, it undergoes a pressure-volume change - an isochoric process typical to closed systems.
Another everyday example is a sealed pot of boiling water. Here, heat energy is transferred into the pot, altering the state of water from a liquid to a gas (steam). However, since the pot is sealed, no mass escapes the system, illustrating a classic example of a closed system in thermodynamics.
Applications of Closed Systems in Thermodynamics
In the realm of thermodynamics, the concept of a closed system is of central importance. Understanding its applications can help you, as an aspiring engineer, devise solutions that improve the efficiency of various technologies and industrial processes.
Practical Applications of Closed Systems in Thermodynamics
Applying the principles of closed systems in thermodynamics spans numerous sectors, including but not limited to, power generation, refrigeration, automotive engineering, and countless industrial processes.
Power Generation: In power plants, closed system thermodynamics is used to harness energy. For example, in a Rankine cycle - commonly used in power plants, the working fluid is recycled continuously in a closed loop. The system absorbs heat energy and converts it into mechanical work, ultimately producing electricity.
\[ \Delta U = Q - W \]
You can use this equation to evaluate the performance and efficiency of the power plants.
Refrigeration and Air Conditioning: The operations of refrigeration and air conditioning systems are textbook examples of closed systems. The refrigerant flows in a closed cycle, where it undergoes phase changes to remove heat from the designated area.
Automotive Engineering: An internal combustion engine is a classic closed system example. The explosion of fuel in the cylinder, captured in a closed space, generates power that drives your vehicles.
The operation of this engine can be broken down into a sequence of thermodynamic processes – primarily isochoric (constant volume) and adiabatic expansions and compressions.
Industrial Processes | Industrial procedures such as chemical reactions in a closed container, distillation, and phase changes also fall under the purview of closed system thermodynamics. These processes can be modelled and optimised using thermodynamic principles. |
The applications above give you a glimpse of how pervasive and crucial closed system thermodynamics is in the realms of modern engineering and industrial processes.
Advanced Applications of Closed Systems in Thermodynamics
Some advanced applications of closed systems in Thermodynamics include the designing of spacecraft propulsion systems, supercritical power plants, and innovative energy storage systems.
Spacecraft Propulsion: In spacecraft propulsion, the propulsion system acts as a closed system where the exhaust gases, by expanding and pushing against the walls of the engine, produce the necessary thrust. These processes can be examined in-depth using the principles of closed system thermodynamics.
Supercritical Power Plants: Supercritical power plants operate under high pressures and temperatures, transitioning from liquid to gas states without a definitive boiling point – a situation that presents unique modelling challenges. Thermodynamics of the closed system significantly aids in designing and optimising such power plants.
Energy Storage Systems : Closed system thermodynamics is also vital for designing energy storage systems such as pumped hydro storage and compressed air energy storage, where energy conservation principles are critical.
In all these applications, using the principles of closed system thermodynamics, you can analyse and optimise the energy transferral processes, thereby holding the potential for enhancing efficiencies and advancements in the technology.
In conclusion, understanding and applying closed system thermodynamics has significant implications in enhancing the efficiency, performance, and overall feasibility of various systems across numerous industries.
Closed Systems in Thermodynamics – The Formula
In thermodynamics, the concept of closed systems is governed by a specific formula, originating from the First Law of Thermodynamics. This law is essentially a statement of energy conservation which, for a closed system, can be expressed as:
\[ \Delta U = Q - W \]
Interpreting the Closed System Thermodynamics Formula
To understand the closed system thermodynamics formula, it is imperative to break down its constituents, each representing a particular energy transaction.
- \(\Delta U\) illustrates the internal energy change of the system.
- \(Q\) represents the net heat transfer - heat absorbed by the system from its surroundings.
- \(W\) symbolises the work done by the system on its surroundings.
The formula essentially encapsulates how energy in the form of heat (\(Q\)) and work (\(W\)) alters the system's internal energy (\(\Delta U\)).
For a closed system, \(\Delta U = Q - W\) is the fundamental equation. This equation states that the net heat supplied to the system minus the work done by the system is equal to the change in internal energy.
Let's consider a scenario where a system is subjected to an amount of heat \(Q\) while it does work \(W\) on its surroundings.
If the system absorbs heat, \(Q\) is positive. Conversely, if the system loses heat or work is done on the system, \(W\) is negative. These conventions follow the physics sign conventions – work done by the system and heat added to the system are positive, and vice versa.
Therefore, the change in internal energy, \(\Delta U\), can be increased by adding heat to the system or doing work on it, and it can be reduced by removing heat from the system or letting the system do work.
Understanding this formula and the science it embodies forms the bedrock of thermodynamic analysis and is crucial to guide experimental procedures accurately.
Application of the Closed System Thermodynamics Formula
The closed system thermodynamic formula is instrumental in numerous applications. Power generation, cooling systems, engines, and industrial processes - all use this formula for optimisation and efficiency improvements.
In power generation, the formula can be used to calculate the thermal efficiency of a power plant by quantifying the internal energy change and measuring the work output.
For cooling systems like refrigerators and air conditioners, the formula can be utilised to calculate the coefficient of performance (COP), thereby evaluating the system's effectiveness.
The internal combustion engines that power most cars apply the thermodynamic formula during each cycle of operation. By altering the mechanics of this operation, improvements to fuel efficiency and power output can be obtained.
Lastly, various industrial processes apply the formula to enhance the efficiency of chemical reactions. A fundamental understanding of the formula allows engineers to leverage thermodynamic principles to optimise heat exchange, carry out accurate mass balance analyses, and much more.
To summarise, the formula for closed system thermodynamics, \(\Delta U = Q - W\), informs much of the day-to-day applications in the field of engineering and beyond. Whether you are an engineer, applied physicist, or environmental scientist, this formula will always be your guide in understanding and manipulating the invisible energy flows around us.
Exploring Open and Closed Systems in Thermodynamics
In the universe of thermodynamics, the concepts of open and closed systems stand as two pillars that shape our understanding of how energy and matter interact and transform. Getting to grips with these structures can be really advantageous for students like you in mastering thermodynamics.
Contrasting Open and Closed Systems in Thermodynamics
Open systems and closed systems are foundational elements in studying thermodynamics. These are theoretical constructs that help you comprehend the behaviour of systems under various conditions. They are defined based on the exchange of matter and energy with the surrounding environment.
An open system refers to a system where both matter and energy can transfer between the system and its surroundings. This exchange of energy can occur in the forms of heat, work, or both. On the other hand, mass can enter or leave the open system. As an everyday example, imagine a cup of hot coffee left on a table. The system (coffee) loses heat to the surroundings, and it can receive dust particles from the environment.
A closed system refers to a system which allows the transfer of energy (heat and work) but does not allow the transfer of mass with the outside environment. To visualise this, think of a sealed thermos with hot liquid. The content can transfer heat through the thermos walls but does not allow any transfer of matter in and out of it.
Using these definitions as the premise, thermodynamic analyses can be classified based on whether they deal with open or closed systems. This forms the basis of different branches of thermodynamics: Classical Thermodynamics primarily focuses on closed systems, whereas Fluid Mechanics and Heat Transfer primarily deal with open systems.
There is also a third category known as an isolated system which does not allow either matter or energy to exchange with its surroundings. Such systems are purely theoretical as perfectly isolated systems do not exist in nature.
Applications and Examples of Open vs Closed Systems in Thermodynamics
There are a multitude of real-life examples and applications for both open and closed systems. Here, we will delve into them in more detail to better understand their practical relevance.
Open Systems: A boiling pot on a stove is an example of an open system. The system (water in the pot) gains heat from the burner (surroundings) and also allows mass (steam) to escape into the surrounding environment. Another magnificent example is a car radiator, an open system where coolant absorbs heat from the engine and exchanges heat (usually loses) with the surrounding air.
A commercial aircraft cruising at high altitude can also be described as an open system. As it consumes fuel (a decrease in mass), it simultaneously produces thrust by expelling hot gases (also a change in mass) while also exchanging heat with its environment.
Closed Systems: On the other hand, let's take a pressure cooker as an example of a closed system. Once sealed, the pressure cooker does not allow mass to cross its boundaries, but it does allow for the transfer of heat from the stove's flame.
Another example is the internal combustion engine of a car. During combustion, mass is not exchanged with the surroundings, but heat is transferred by the working fluid. As the fuel and air inside the engine are ignited, they release energy that is transferred to the piston in the form of work, which then helps to move the car.
To conclude, the concepts of open and closed systems are elemental to understanding thermodynamics. By contrasting their properties and examining their applications, you can gain a profound understanding of their roles in various physical, chemical, and engineering phenomena. As you further explore thermodynamics, these concepts will serve as vital tools in your scientific analysis and problem-solving toolkit.
Closed System Thermodynamics Problems and Solutions
If you're studying engineering, physics, or a related discipline, you're likely to encounter numerous problems related to closed system thermodynamics. These problems require not only a thorough understanding of the underlying principles, but also an ability to practically apply these principles to real-world scenarios.
Case Studies on Closed System Thermodynamics
There is a myriad of case studies stemming from the closed system thermodynamics domain. These cases illustrate variations in heat exchange, work transfer, and changes in internal energy. They serve to examine thermodynamics in practical contexts, providing valuable insight into resolving complex closed system scenarios.
Here are a couple of illustrative examples:
Case Study 1: Consider a tank containing a certain amount of inert gas, and the tank is being heated. The tank, in this case, acts as a closed system. As heat is supplied, the internal energy of the gas (\(\Delta U\)) increases – this increase must be calculated. The first problem here is balancing the heat exchange. Assuming the tank is perfectly insulated (i.e. no heat loss), the entire heat supplied will contribute to the increase in internal energy and can be calculated using the formula \(Q = nC\Delta T\), where \(n\) is the number of moles, \(C\) is the specific heat at constant volume, and \(\Delta T\) is the change in temperature.
Case Study 2: Picture a closed system of a gas inside a piston-cylinder device that is expanding against the atmospheric pressure. As the gas expands, it performs work on its surroundings by pushing the piston outward. This is an example of a closed system that does work on its surroundings. The work done can be calculated using the generalized work equation \(W = P\Delta V\), where \(P\) stands for pressure and \(\Delta V\) is the change in volume.
These case studies elucidate how fundamental thermodynamic principles apply to real-world situations. Working through these problems helps you better comprehend the principles of thermodynamics and their applications.
Practical Solutions to Closed System Thermodynamics Problems
Having looked at some common problems relating to closed systems in thermodynamics, let's now discuss their solutions in more detail.
Solution for Case Study 1: Here, the heat supplied to the gas causes the internal energy (\(\Delta U\)) to increase. This increase in internal energy can be calculated by rearranging the first law of thermodynamics formula \(\Delta U = Q - W\). Since there's no work being done (the gas neither expands nor compresses), \(W=0\). Hence, the entire heat supplied \(Q\) contributes to the increase in internal energy. We then can compute \(Q\) using the formula \(Q = nC\Delta T\), and this value gives us the increase in internal energy \(\Delta U\). Here, \(n\) represents the number of moles of gas, \(C\) is the specific heat capacity of the gas, and \(\Delta T\) is the change in the gas's temperature.
Solution for Case Study 2: In this example, the gas's expansion performs work on the surroundings by moving the piston.The work done by the system is given by the formula \(W = P\Delta V\). Since the pressure \(P\) is atmospheric pressure, and \(\Delta V\) is the change in volume, \(W\) can be calculated readily. However, this work done comes at the cost of the internal energy (\(\Delta U\)). Hence, if there is no exchange of heat with the surroundings (an adiabatic process), this work done is exactly equal to the decrease in internal energy, according to the first law of thermodynamics (\(\Delta U = Q - W\), but \(Q=0\)).
Arriving at these solutions involves comprehending the problem, identifying the relevant thermodynamics principles, and then applying the appropriate formulas or algorithms. Mastery of this process not only provides deeper insights into the principles of thermodynamics but also cultivates robust problem-solving skills that are highly applicable across scientific and engineering disciplines.
Closed System Thermodynamics - Key takeaways
- Definition of Closed Systems in Thermodynamics: A closed system refers to a system which permits the transfer of energy (heat and work) but does not allow the transfer of mass with the outside environment.
- Examples of closed systems: Pressurized aerosol can, internal combustion engine, and a sealed pot of boiling water.
- Applications of closed system thermodynamics: Power plants such as Rankine cycle, refrigeration and air conditioning, internal combustion engines and numerous industrial processes including chemical reactions in closed containers and distillation.
- Closed System Thermodynamics Formula: \(\Delta U = Q - W\), which represents the First Law of Thermodynamics. \(\Delta U\) represents the change in internal energy of the system, \(Q\) represents the net heat transfer - heat absorbed by the system from its surroundings and \(W\) represents the work done by the system.
- Contrasting Open and Closed Systems: An open system allows the transfer of both matter and energy while a closed system allows transfer of energy but not matter. An isolated system doesn't permit either matter or energy to exchange with its surroundings.
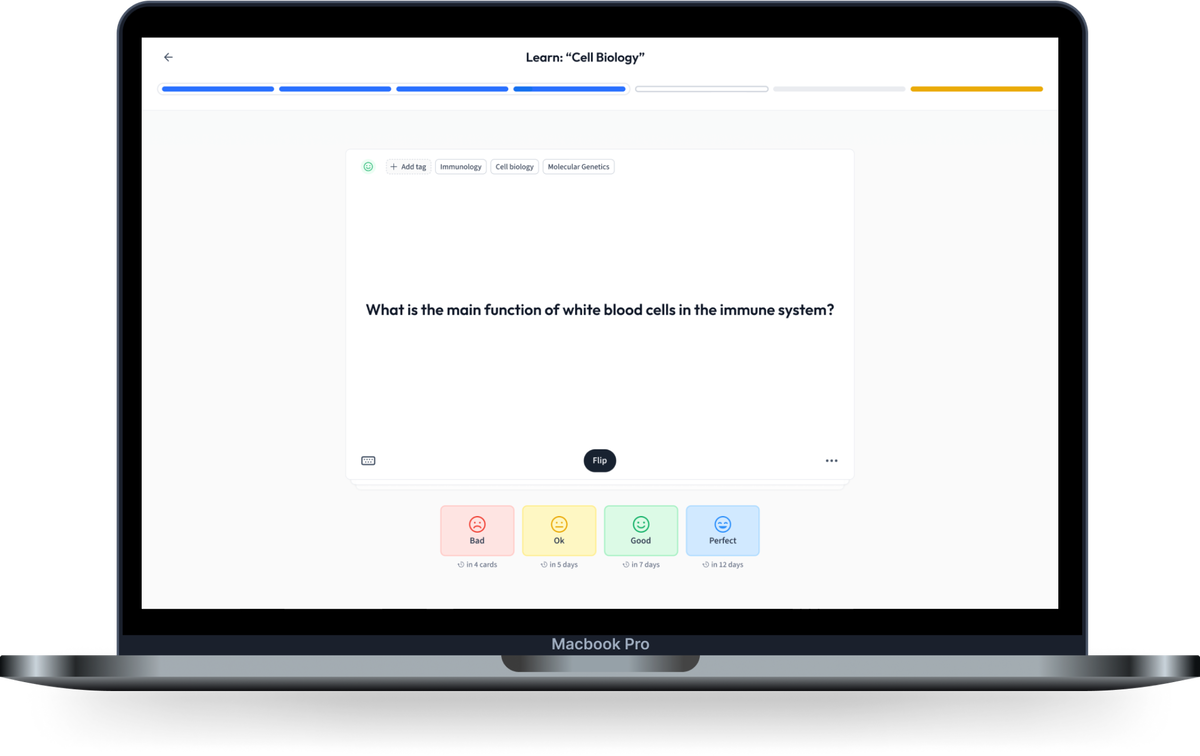
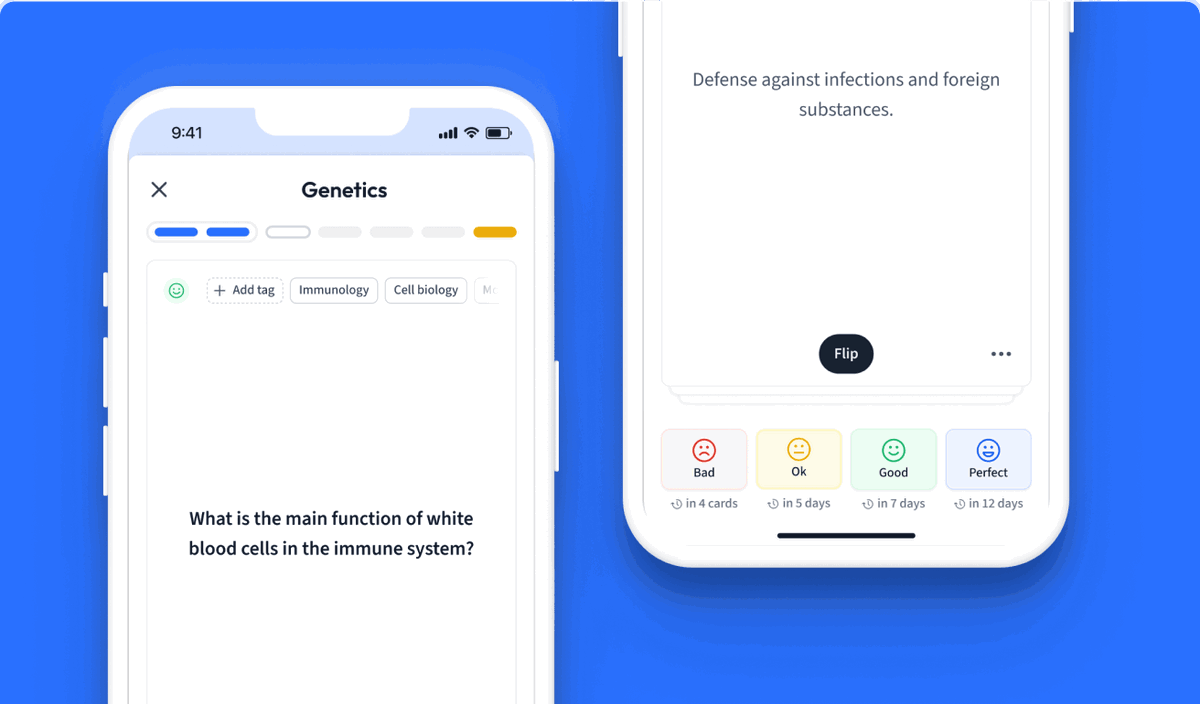
Learn with 158 Closed System Thermodynamics flashcards in the free StudySmarter app
We have 14,000 flashcards about Dynamic Landscapes.
Already have an account? Log in
Frequently Asked Questions about Closed System Thermodynamics
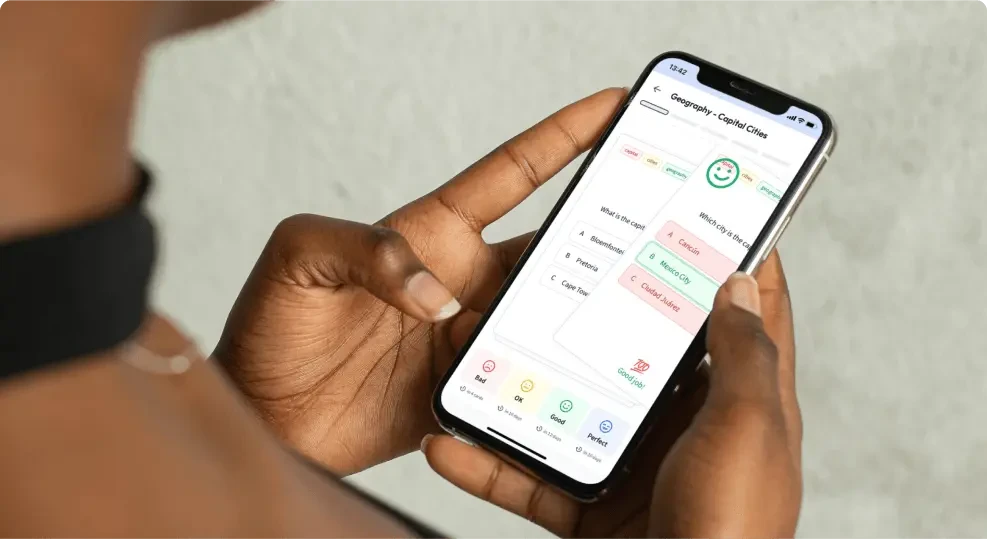
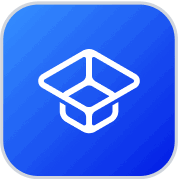
About StudySmarter
StudySmarter is a globally recognized educational technology company, offering a holistic learning platform designed for students of all ages and educational levels. Our platform provides learning support for a wide range of subjects, including STEM, Social Sciences, and Languages and also helps students to successfully master various tests and exams worldwide, such as GCSE, A Level, SAT, ACT, Abitur, and more. We offer an extensive library of learning materials, including interactive flashcards, comprehensive textbook solutions, and detailed explanations. The cutting-edge technology and tools we provide help students create their own learning materials. StudySmarter’s content is not only expert-verified but also regularly updated to ensure accuracy and relevance.
Learn more