Understanding the Compressor in Engineering Thermodynamics
Within the field of engineering thermodynamics, a prime component that you often come across is the compressor. In essence, a compressor is a mechanical device that increases the pressure of a gas or air by reducing its volume. This plays a crucial role in many engineering processes, especially in power generation and refrigeration systems.
Compressor: Deep Dive into its Meaning
A compressor works on the principle of thermodynamics, specifically the ideal gas law, which can be expressed as \(PV = nRT\), where \(P\) is pressure, \(V\) is volume, \(n\) is the number of moles of gas, \(R\) is the gas constant, and \(T\) is temperature. Here, the compressor, by decreasing the volume \(V\), results in an increase in pressure \(P\) since the right-hand side of the equation remains constant.
Compressor: A mechanical device that reduces the volume of gas or air, therefore, increases its pressure. It's a key component in thermodynamic processes.
There are two broad types of compressors based on their mechanism of compression: positive displacement and dynamic compressors.
- Positive Displacement Compressors: They work by taking in a certain amount of air in a chamber and physically reducing the volume of the chamber.
- Dynamic Compressors: They work by accelerating the air to high speed and then decelerating it to increase pressure.
Exploring Different Compressors Examples in Engineering
Within the realm of engineering, quite a few types of compressors have been designed to cater to diverse requirements.
Type of Compressor | Application |
Reciprocating Compressor | Petrochemical plants, oil refineries |
Rotary Screw Compressor | Powering high power air tools |
Centrifugal Compressor | Aircraft engines, large refrigeration systems |
For instance, a Reciprocating Compressor, which falls under the category of positive displacement compressors, is widely used in petrochemical plants and oil refineries. They work by a piston moving back and forth in a cylinder, compressing the gas with each stroke.
Practical Compressors Applications in Thermodynamics
Delve into the handy application of compressors in day-to-day life. They're especially significant in power generation and refrigeration systems. Here's how:
- In power generation, steam is often condensed into water using a compressor, making it more efficient and eco-friendly.
- In refrigeration systems, compressors play a vital role in the cooling cycle. They compress the refrigerant, raising its temperature. The refrigerant then flows through the condenser, releasing heat and cooling down as it transforms into a high-pressure liquid.
Interestingly, the principle of a compressor is also used in jet engines of aircraft. Here, the air is sucked in and compressed to a high pressure before it is combusted with fuel. The high-pressure combustion gases then produce thrust to propel the aircraft forward.
Thus, your understanding of compressors as essential elements in various thermodynamic processes becomes critical while delving into engineering dynamics.
Maths Behind the Compressor: The Formulas
In the realm of engineering thermodynamics, the mathematics that governs the operation of compressors is a fundamental aspect. Understanding this aids in comprehending machine efficiency, enhances the problem-solving skills on various thermodynamic problems, and insights into how the compressors work, specifically in real-world scenarios.
Unlocking the Compressors Formula: A Complete Breakdown
The operation of a compressor can be well explained using the ideal gas law. At a constant temperature, if the volume of gas decreases, the pressure increases and vice versa, as expressed by the relation \(PV = nRT\).
Ideal Gas Law: A fundamental principle in thermodynamics, indicating that the product of pressure and volume of gas remains constant at a fixed temperature, represented by the formula \(PV = nRT\).
All real gases obey this law at low pressures and high temperatures. It is the relationship between the pressure, volume and temperature of a gas. Most compressors work on this principle as they decrease the volume, causing the pressure to increase.
Actual and Ideal Processes of Compressors
In compressors, both actual and ideal processes occur. Ideal process is an isentropic process, where the process takes place at a constant entropy. However, in actual processes, there are inefficiencies leading to an increase in entropy. The efficiency of a compressor, also known as isentropic efficiency, is the ratio between the work done in the actual process to the work done in the ideal or isentropic process.
Isentropic Efficiency: It's a measure of deviation of the actual compressor process from the ideal process. Expressed as the ratio of work done in the actual process to the work done in the ideal process.
Compressor Efficiency Thermodynamics: Decoding the Calculation
Compressor Efficiency: In thermodynamics, it's measured by isentropic efficiency. Higher the isentropic efficiency, closer the real cycle to the ideal cycle, and hence better the compressor.
When calculating isentropic efficiency of a compressor, we use the formula:
\[ \eta_{isentropic} = \frac{Work_{isentropic}}{Work_{actual}} \]Here, the isentropic work is less than the actual work. The isentropic process is a reversible and adiabatic process where there is no heat transfer, while the actual process involves heat transfer, increasing the total amount of work done, thus, reducing the efficiency.
Implementing Compressors Formula in Real World Scenarios
In real-world scenarios, compressors play a pivotal role in countless applications. From fridges, air conditioners, and manufacturing industries to jet engines, the underlying principle remains the same.
For instance, in the refrigeration industry, the operation of a fridge involves a compressor that operates by the principles discussed, using the ideal gas law. The compressor compresses the refrigerant, which increases its pressure and temperature. The refrigerant then releases its heat through the condenser coils, becomes cool and expands, thus, decreasing its pressure.
Similarly, in the automotive industry, auto-ignition engines use a compressor to increase the air pressure before it is combusted with petrol. This increased pressure leads to a more efficient combustion process, contributing to better fuel efficiency of the engine.
In jet engines, the operation begins with air intake at the compressor, where air is compressed and fed into the combustion chamber at high pressure. The compressed air is then mixed with fuel and ignited. The high-pressure combustion gases flow out through the nozzle, propelling the aircraft. This entire process of a jet engine operation, beginning with the compressor, refers back to the integral formulas of compressor thermodynamics.
Thus, the formulas and principles of compressor thermodynamics have practical applications in various fields, providing effective solutions to real-world problems.
An Exploration of Different Types of Compressors
A deep dive into the sphere of engineering thermodynamics reveals a sheer diversity of compressors. From Reciprocating Compressor to Rotary Compressor, each type serves varied industrial needs, abiding by the basic principle of volume reduction and pressure increment.
Reciprocating Compressor Thermodynamics: A Detailed Examination
Setting the benchmark in various industries, Reciprocating Compressors are identified by their distinctive piston-cylinder arrangement. They operate on simple thermodynamic principles and deliver highly pressurised gas output.
Bearing semblance to a motor car engine, a Reciprocating Compressor's central components include a piston, a cylinder, and a system of valves. As the piston reciprocates, or moves back and forth within the cylinder, it alternately sucks in gas and compresses it. This mechanism leverages the basic principle of thermodynamics: as the volume of gas decreases (due to the piston's upward movement), its pressure increases consequentially.
This compressive action obeys the ideal gas law: \(PV = nRT\), where \(P\) represents pressure, \(V\) signifies volume, \(n\) is the number of gas moles, \(R\) is the ideal gas constant, and \(T\) represents the absolute temperature. Here, \(n\), \(R\) and \(T\) remain constant, causing \(P\) to rise and \(V\) to fall. Hence, the Reciprocating Compressor's operation truly captures the spirit of thermodynamics.
These compressors are simple in design, with their piston-cylinder mechanism being straightforward to understand. Popular in industries from petrochemical plants to oil refineries, they are valued for their robustness, flexibility, and ability to handle high pressures.
Comparing Different Compressors: Reciprocating, Rotary, and More
Alongside the Reciprocating Compressor, numerous other compressors cater to the diverse needs of the engineering world, including the Rotary Compressor, Centrifugal Compressor, and Axial Flow Compressor.
The Rotary Compressor employs a different mechanism than its reciprocating counterpart. It uses two rotors, twisted together like a pair of DNA strands. As the rotors turn, the gas trapped between them gets compressed and discharged. Owing to the continuous rotational movement, Rotary Compressors ensure steady airflow, rendering them ideal for powering high power air tools.
On the other hand, the Centrifugal Compressor relies on a dynamic principle whereby kinetic energy gets converted into potential energy. In operation, the gas is accelerated through an impeller's high-speed rotation. It's then slowed down, causing the gas pressure to rise. Centrifugal Compressors, due to their proficiency with large gas volumes and high efficiency, are commonly used for large refrigeration systems and aircraft engines.
Meanwhile, the Axial Flow Compressor, like the Centrifugal Compressor, uses dynamic action. However, it handles gas compression differently. Here, the compressor uses a series of rotating and stationary blades to continuously compress the incoming air. It's an integral part of jet propulsion, contributing to an engine's high speed and efficient operation.
Ups and Downs of Various Compressors in Engineering Thermodynamics
When choosing a compressor, considering the various upsides and downsides of each type is critical. Thus, a comparison table becomes imperative:
Compressor Type | Upsides | Downsides |
Reciprocating Compressor | Robust, flexible, ideal for high-pressure tasks | High maintenance, potential for vibration and noise |
Rotary Compressor | Steady airflow, quiet operation, low maintenance | Struggles at high pressure, not suitable for large volumes |
Centrifugal Compressor | Handles large volumes, high efficiency, low maintenance | Complex design, initial cost is high |
Axial Flow Compressor | High efficiency at high speed, handles large volumes | Complicated design, suited only for specific applications |
Understanding this table equips you in making an informed choice, considering your project's specific requirements. For high-pressure tasks, you might lean towards the reciprocating variant, whereas for handling larger volumes, centrifugal or axial flow compressors might be your go-to.
Thus, each type of compressor, despite their downsides, holds their own in the field of engineering thermodynamics, charting extensive applications and contributing significantly to the engineering landscape.
Enhancing Compressor Efficiency in Thermodynamics
In the realm of thermodynamics, compressor efficiency holds profound importance. A highly efficient compressor utilises energy in an optimal manner and ensures effective operation in various applications. However, achieving optimum efficiency necessitates meticulous efforts to boost the compressor's functional dynamics.
Step by Step Guide to Improve Compressor Efficiency Thermodynamics
Achieving maximum compressor efficiency involves implementing a series of strategic actions that work towards reducing energy loss, boosting output, and ensuring sustained operational performance. Here's a detailed step-by-step guide to enhance your compressor's thermodynamic efficiency:
- Optimisation of Operating Conditions: Firstly, the operating conditions of the compressor need to be optimised. This involves maintaining adequate pressure levels, ensuring proper compression ratio and minimising heat rejection, all of which aid in reducing energy wastage and boosting efficiency.
- Regular Monitoring: Regularly monitor the compressor's vital signs such as inlet air temperature and compression ratio. Explicitly, tracking the compressor's 'pressure ratio' against the 'isentropic efficiency' helps to identify potential performance issues and helps facilitate corrective action.
- Reducing Compressor Discharge Temperature: A high compressor discharge temperature induces more heat into the compressor system, thereby reducing efficiency. Implementing efficient cooling systems, thermally insulating discharge lines, and regulating the compression ratio can help control discharge temperature effectively.
- Maintenance Regime: Ensuring a robust maintenance regime is indispensable. Regular servicing, replacement of worn out parts, and a check on oil levels can significantly enhance compressor efficiency.
- Capitalising on Heat Recovery: A significant fraction of energy consumed in compressors is wasted as heat. Deploying a heat recovery system can enable utilising this waste heat for space heating or water heating, indirectly improving the overall energy efficiency.
The rate of heat transfer during compression and expansion can be calculated using the formula:
\[ Q = m \cdot Cp \cdot (T2 - T1) \]where \( m \) is the mass flow rate of the gas, \( Cp \) is the specific heat at constant pressure, and \( T2 \) and \( T1 \) are the final and initial temperatures respectively.
In conclusion, enhancing compressor efficiency in thermodynamics is a systematic process that mandates diligent execution of strategies and ensuring optimum operational parameters. By adhering to the recommended steps, you can perpetually improve the performance and efficiency of compressors.
Why is Compressor Efficiency Crucial in Thermodynamics?
Thermodynamic efficiency of compressors is the ratio of work output to the work input, and it plays a paramount role in determining the performance of a compressor. An efficient compressor is synonymous with effective energy use and sustainability. Here are some reasons why compressor efficiency is vital in thermodynamics:
- Economic Sustainability: An efficient compressor utilises less energy to achieve the same level of output, translating into significant cost savings over time. By saving on energy costs, industries can improve their economic sustainability in the long run.
- Environmental Impact: With climate change being a pertinent issue, achieving higher compressor efficiency can minimise carbon footprints and contribute to better environment sustainability. A more efficient compressor consumes less energy, thereby reducing greenhouse gas emissions.
- Productivity: High-efficiency compressors enhance overall productivity. They produce high-quality air at a greater volume, enabling machines and tools to perform their functions more effectively, leading to higher overall productivity in industries.
- Reliability: An efficient compressor runs more smoothly, ensuring fewer breakdowns and higher reliability and endurance. This is particularly crucial in industries where compressed air forms the backbone of operations.
- Longevity: Compressors that operate efficiently display less wear and tear over time, extending their operational life and reducing the frequency and cost of replacements, thereby increasing their lifespan.
Compressor efficiency can be calculated using the ratio of the isentropic power to the brake power of the compressor:
\[ \eta_{compressor} = \frac{Power_{isentropic}}{Power_{brake}} \]\( \eta_{compressor} \) is the compressor efficiency, \(Power_{isentropic} \) is the isentropic power, and \(Power_{brake} \) is the brake power of the compressor. The brake power is the actual power required by the compressor while the isentropic power is the ideal power that will be required if the process were isentropic.
Therefore, achieving a high compressor efficiency is crucial to ensure the smooth running of industrial systems. Whether it's the economic and environmental aspects or the productivity, reliability, and longevity of operations – compressor performance can profoundly determine the overall success of an application. Always remember: The journey of enhancing compressor efficiency is a relentless one, with the rewards reaped across economic, environmental, and efficiency spheres.
Potential Challenges and Solutions in Compressors
Compressors form the heart of thermodynamic systems, and it's essential to understand the challenges these vital components might face and probe into potential solutions. From mechanical issues to operational inefficiencies, compressor issues encompass a gamut of possibilities, each requiring a distinct troubleshooting approach.
Dealing with Common Issues in Compressors: A Practical Guide
Several common problems plague compressors in their lifetime. These issues can hamper the compressor's performance and efficiency. The solutions range from simple fixes to complex troubleshooting. Highlighted below are key problems, detection methods, and potential solutions:
- Overheating: Overheating is a frequent issue in compressors. It can be identified through an increase in the compressor's discharge temperature. Overheating can be caused by factors such as inadequate cooling or improper compression ratio. The solution lies in maintaining a proper compression ratio, improving the cooling mechanism, and regularly monitoring the compressor discharge temperature.
- High Vibrations: Vibrations often indicate mechanical issues in the compressor. It can be due to worn-out bearings, misalignment of parts, or imbalances in rotating components. Regular monitoring and maintenance can ward off such issues. The parts causing the vibrations can often be identified and replaced or repositioned to reduce the vibrations.
- Pressure Fluctuations: Pressure fluctuations can lead to inefficient operation of the compressor. An increase in pressure can signify clogged air filters or faulty valves. Regular cleaning and replacement of these components can keep pressure fluctuations in check.
Furthermore, investing in technology that provides real-time data about compressor status can help detect issues in advance and mitigate problems promptly. For instance, condition monitoring systems can facilitate speedy issue detection and resolution.
Innovative Solutions to Compressor Challenges in Engineering Thermodynamics
Engineering thermodynamics caters to the efficient utilisation and conversion of energy. Compressors play a central role, and modern technology offers some next-gen solutions to compressor challenges. Some of these cutting-edge interventions include:
- AI-Enabled Diagnostics: Artificial Intelligence (AI) and Machine Learning can be leveraged to predict and diagnose compressor issues at an early stage. These technologies can analyse patterns from historical data and raise alarm for potential faults, even before they occur, facilitating proactive maintenance and avoiding unforeseen downtime.
- Eco-Friendly Alternatives: Eco-friendly compressors are a step towards environmental sustainability. For instance, water-injected screw compressors operate without oil, reducing the environmental impact. Using alternatives that minimise carbon emissions and promote sustainability is a forward-thinking solution to compressor issues.
- Internet of Things (IoT) in Compressors: IoT can provide real-time data about the compressor's functioning and efficiency. This information can help keep track of the compressor's status and provide valuable insights into the system's repair and maintenance needs.
By taking an innovative approach to tackle compressor issues, these futuristic technologies expand the horizons of possibilities. Remember, enhancing compressor efficiency and tackling challenges is a dynamic process - ever-evolving with technological advancement. With the right approach and utilisation of modern tech, navigating compressor challenges becomes an achievable feat that paves the way to efficient and optimal system operations.
Compressor - Key takeaways
- Compressor: A device that decreases volume of gas causing its pressure to increase. Compressors work based on the principles of the ideal gas law: \(PV = nRT\).
- Ideal and Actual Compressor Processes: Ideal process is an isentropic process with constant entropy. Actual processes have inefficiencies leading to entropy increase. The ratio of work done in the actual process to the ideal process is the compressor's isentropic efficiency.
- Isentropic Efficiency: A measure of deviation of the actual compressor process from the ideal process. In compressor efficiency thermodynamics, it is calculated using the formula: \(\eta_{isentropic} = \frac{Work_{isentropic}}{Work_{actual}}\).
- Compressors in Real-World Scenarios: Compressors have applications in industries like refrigeration, automotive, and aerospace, where they operate based on the principles of the ideal gas law and compressor thermodynamics.
- Types of Compressors: Different types of compressors like Reciprocating Compressor, Rotary Compressor, Centrifugal Compressor, and Axial Flow Compressor cater to different needs in the engineering world based on principles of volume reduction and pressure increment.
- Reciprocating Compressor Thermodynamics: Reciprocating Compressors work based on simple thermodynamic principles using piston-cylinder arrangement, using the principle of the ideal gas law \(PV = nRT\).
- Compressor Efficiency Thermodynamics: Compressor Efficiency is important for optimal energy use and effective operation. It is enhanced by implementing strategies to reduce energy loss, boost output, and ensure sustained operational performance.
- Importance of Compressor Efficiency in Thermodynamics: Compressor Efficiency contributes to economic sustainability, environmental impact, productivity, reliability, and longevity in industrial operations, and can be calculated using \(\eta_{compressor} = \frac{Power_{isentropic}}{Power_{brake}}\).
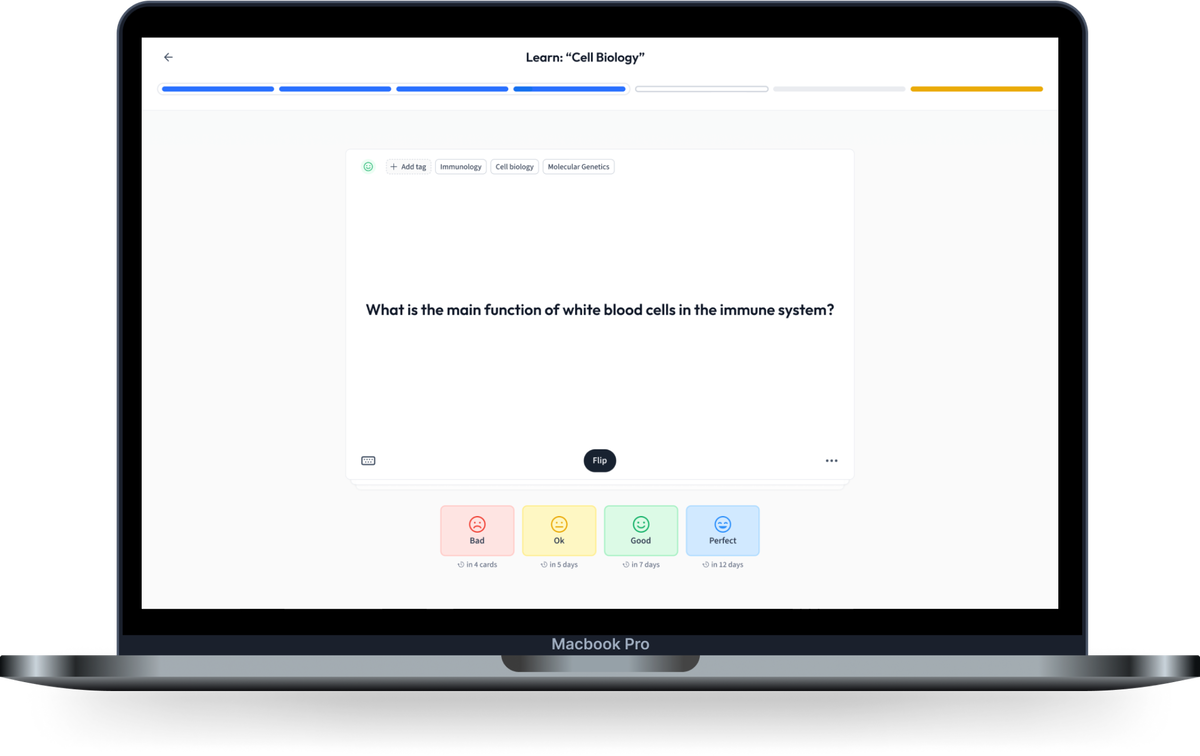
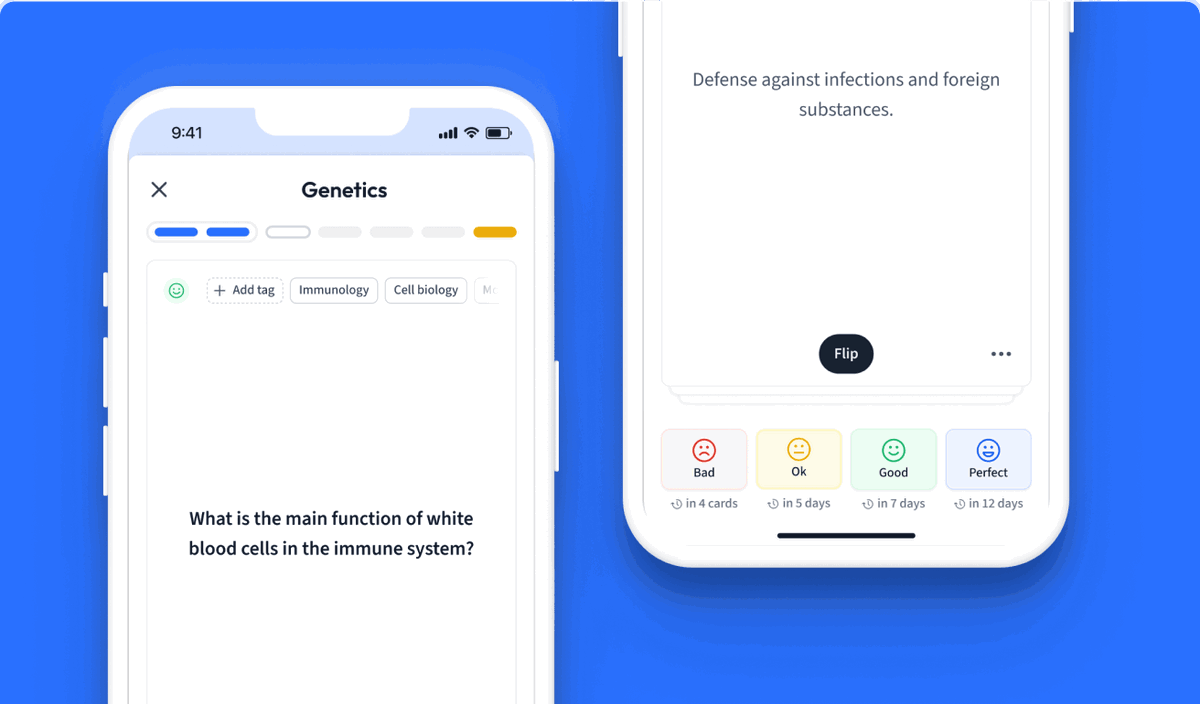
Learn with 15 Compressor flashcards in the free StudySmarter app
We have 14,000 flashcards about Dynamic Landscapes.
Already have an account? Log in
Frequently Asked Questions about Compressor
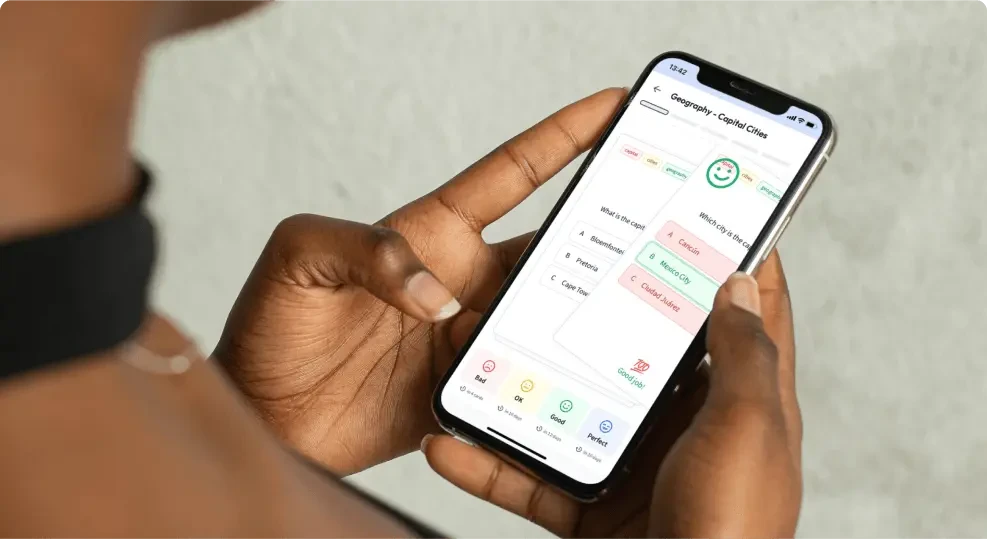
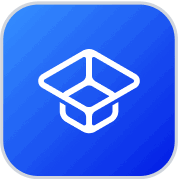
About StudySmarter
StudySmarter is a globally recognized educational technology company, offering a holistic learning platform designed for students of all ages and educational levels. Our platform provides learning support for a wide range of subjects, including STEM, Social Sciences, and Languages and also helps students to successfully master various tests and exams worldwide, such as GCSE, A Level, SAT, ACT, Abitur, and more. We offer an extensive library of learning materials, including interactive flashcards, comprehensive textbook solutions, and detailed explanations. The cutting-edge technology and tools we provide help students create their own learning materials. StudySmarter’s content is not only expert-verified but also regularly updated to ensure accuracy and relevance.
Learn more