Understanding Regenerative Rankine Cycle
The Regenerative Rankine Cycle is primarily used in power generation to recuperate heat that would have otherwise been wasted in a simple Rankine Cycle. This enhances the overall efficiency of the system.
The Meaning of Regenerative Rankine Cycle
The concept of the Regenerative Rankine Cycle (RRC) plays an integral part in how power plants – particularly ones that rely on steam power – operate. The term 'regenerative' is used because this cycle incorporates a method to recover heat during the cycle.
It's interesting to note that the idea of reusing heat isn't novel. This brilliant thermo-dynamic principle has been used for centuries, particularly in steam-based power plants. It significantly lessens the quantity of heat that is expelled to the environment, ensuring more efficient use of fuel.
The Principle Behind the Regenerative Rankine Cycle
Relatively more specific, the Regenerative Rankine Cycle deviates from a simple Rankine Cycle by using feedwater heaters. These heaters recover energy from the steam exiting the turbines. This process, known as 'regeneration', is the reason for the cycle's name as it regenerates heat internally, hence bettering the efficiency of the cycle and generating more power with the same amount of input.
For instance, consider boiling water on a stove. Some of the heat escapes into the surrounding environment, which is wasted energy. In a regenerative cycle, however, steps are taken to reduce this heat loss.
The Components of the Regenerative Rankine Cycle
The primary components of the Regenerative Rankine Cycle include the boiler, turbine, condenser, and pump, along with one or multiple feedwater heaters. Let us delve a bit deeper into each element:
- Boiler: This is where heat (from burning fuel) is added to the water to swap it into steam, always under high pressure.
- Turbine: The steam from the boiler is expanded here, turning the turbine to create electrical energy.
- Condenser: The exhaust steam from the turbine is cooled and condensed back into water.
- Pump: The water from the condenser is then pumped back into the boiler to complete the cycle.
- Feedwater Heaters: They capture and utilize the remaining heat from the low-pressure turbine exhaust steam to pre-warm the water before it goes back into the boiler, enhancing the efficiency further.
A feedwater heater is a power plant component which pre-warms water that is fed back into the boiler, using heat from the turbine exhaust steam to increase the thermal efficiency of the cycle.
Here is a simple table showing the step by step progress in a Regenerative Rankine Cycle:
Step | Action |
1. | Water is pumped into the boiler. |
2. | Water changes to high-pressure steam in the boiler. |
3. | The steam expands and turns the turbine. |
4. | The exhaust steam is cooled and becomes water. |
5. | The water is heated in feedwater heaters. |
6. | Heated water returns to the boiler. |
Working with Regenerative Rankine Cycle Examples
An understanding of the Regenerative Rankine Cycle's functionality can be greatly assisted by practical examples. These examples will not only illustrate the overall mechanic but proffer a deep dive into how each component functions within the cycle.
Example One: Exploring the Regenerative Rankine Cycle
Consider a steam power plant operating exclusively on a Regenerative Rankine Cycle. Here, the steam at the exit of the turbine is bled off at various intervals for rejuvenation. This is accomplished through multiple stages of feedwater heating. As such, the heating is accomplished by steam extracted from various stages of the turbine.
Bleeding in this context refers to the removal or diversion of steam from the turbine at a certain stage during the cycle.
Briefly, this process allows the recovery of more heat, resulting in decreased fuel consumption. Also, these diversions of steam significantly increase the overall efficiency of the plant. The actual increase depends on the number of feedwater stages and the conditions at which the heat recovery occurs.
Now, let’s consider a more compound situation. For instance, let's assume the steam has a mass flow rate of 20kg/s at the inlet of the turbine. The steam properties at this point are given as 8MPa, 480°C degrees. It then enters the turbine to expand to an intermediate pressure of 2MPa where a fraction of the steam is bled. There it ultimately expands isentropically to the final pressure of 0.008MPa. Also, pump efficiency of 85% is assumed.
To compute the thermal efficiency of this cycle, the following formula should be utilized:
\[ η_{th} = \frac{W_{net,out}}{Q_{in}} \]Example Two: Regenerative Rankine Cycle in Action
Continuing from the previous example, we can determine the cycle efficiency using the provided data. The total enthalpy is extracted from the boiler until the expanded point of steam. Each specific enthalpy, \( h_{2}, h_{3}, h_{4}, h_{5} \) can be determined from specific pressure and temperature conditions in the given scenario.
Employing a Mollier diagram or steam tables, the enthalpy at every crucial stage can be computed. The net power output (\( W_{net,out} \)) and heat input (\( Q_{in} \)) can thus be garnered. \(\( W_{net,out} \) = W_{turbine} - W_{pump} = m*(h3 - h2) - m*(h5 - h4)\), where m is the mass flow rate and h refers to the specific enthalpy at different stages. The heat input \( Q_{in} \)= m*(h3 - h1), where h1 is the initial state at the boiler entrance.
The computed values then need to be substituted back into the thermal efficiency formula \(η_{th} = \frac{W_{net,out}}{Q_{in}}\) to get the final efficiency of the cycle.
By understanding these methods to calculate the efficiency of a Regenerative Rankine Cycle, you can observe the significant increases in efficiency derived from the use of regeneration contrasted with a standard Rankine Cycle.
Essentially, every Regenerative Rankine Cycle operation hinges on these principles, albeit some variances in the specifics could be noticed in some industrial applications. However, the fundamental idea of recovering and reutilising heat remains the same.
Practical Applications of the Regenerative Rankine Cycle
The Regenerative Rankine Cycle has a myriad of practical applications, particularly in the realm of power and energy production. In any situation where there is a demand for an efficient conversion of heat into work, the Regenerative Rankine Cycle finds its utility. By harnessing the energy that would have otherwise been lost, this cycle increases operational efficiency and promotes resource conservation.
Utilisation of Regenerative Rankine Cycle in Energy Production
The primary application of the Regenerative Rankine Cycle is within energy production sectors where there are extensive requirements for efficient and reliable power generation. In these situations, conservation and optimum use of resources are central tenets for sustainability and economic viability.
Power plants, especially those operating on non-renewable fuels, have adopted the Regenerative Rankine Cycle as a standard functionality. Specifically, in the coal and nuclear power plants, the heat from the fuel and reactor respectively is used to generate steam. This steam, at a high temperature and pressure, is then expanded in a turbine to generate power.
Typically, in a Simple Rankine Cycle, this steam would then be cooled and pumped back to the boiler to restart the cycle. However, this process doesn't extract the full energy potential of the steam. In the Regenerative Rankine Cycle, the steam is bled at different stages and passed through the feedwater heaters. This system thus recovers the latent energy from the steam before it condenses.
The feedwater heater is an integral hardware component of this cycle. Its primary role is to transfer heat from the residual steam to the feedwater.
It's crucial to mention that multiple feedwater heaters can be utilised, each using the bled steam from different stages of the turbine. This staged turbine exhaust heat recovery forms a critical part of the energy recuperative process.
Essentially, here is what occurs:
- When steam is bled from the turbine, its pressure and temperature drop, releasing heat.
- This heat then warms up the water that is about to be pumped back into the boiler.
- The boiler now needs less heat to produce the steam, as the water is pre-heated, consequently improving the overall cycle efficiency.
Regenerative Rankine Cycle in Industrial Settings
In industrial settings, powerful systems are required to enhance efficiency and productivity. The Regenerative Rankine Cycle is prevalent in such arenas due to its energy-saving properties.
Furthermore, the Rankine Cycle and its derivatives are implemented in numerous ways outside the power production sector. Industrial operations routinely harness residual heat from processes and convert it to useful work using the principles of the Regenerative Rankine Cycle.
An example is the combined heat and power plants (CHP), where waste heat from electricity production is used for heating solutions. The waste heat can be transferred directly for heating purposes, or it can be used in a secondary cycle, often a Regenerative Rankine Cycle, to generate additional electricity. By coupling the production of heat and power, these systems typically achieve very high overall energy efficiency.
Moreover, the marine industry leverages the benefits of the Regenerative Rankine Cycle. Large ocean-going vessels have huge engines for propulsion, often running on heavy fuel oil or diesel. The exhaust heat from these engines is substantial, and rather than just venting it to the atmosphere, it is used in a Regenerative Rankine Cycle to generate additional power for on-board use.
Alluded to earlier is the versatility of the Regenerative Rankine Cycle. By harnessing 'waste' heat for additional power generation, the process boosts energy efficiency and promotes sustainable resource use. As its principles are scalable and adaptable to different conditions, different industries across the globe continue to utilize the Regenerative Rankine Cycle for power generation.
Formulating the Regenerative Rankine Cycle
The Regenerative Rankine Cycle is based upon particular thermodynamic principles and is represented mathematically by a set of formulas. These formulas allow you to delve deep into the effectiveness of the cycle by quantifying the efficiency and work output.
The Basic Formula of the Regenerative Rankine Cycle
Let's start with the fundamental formula of the Regenerative Rankine Cycle. Given that it is a thermodynamic cycle, it primarily deals with heat and work transfer. The total heat input to the cycle (\( Q_{in} \)) and work output from the turbine (\( W_{turbine} \)) are the keystones in the basic formula.
The heat input to the Regenerative Rankine Cycle is the total enthalpy extracted from the boiler and the feedwater heaters. Enthalpy, in this context, is the heat content of the steam entering the turbine and can be denoted using the equation:
\[ Q_{in} = m \cdot (h_{3} – h_{4}) \]The variables in the above equation stand for:
- \(m\): Mass flow rate of the steam
- \(h_{3}\): Enthalpy of steam at the end of reheating
- \(h_{4}\): Enthalpy of steam after the feedwater pinch point
Work output from the turbine can be represented as the difference in enthalpies across the turbine, multiplied by the steam mass flow rate. This can be translated into the formula:
\[ W_{turbine} = m \cdot (h_{1} – h_{3}) \]The variables in this equation are:
- \(m\): Mass flow rate of the steam
- \(h_{1}\): Enthalpy of steam at the turbine inlet
- \(h_{3}\): Enthalpy of steam at the end of reheating
These equations provide the basic groundwork for analysing how energy is converted into work in a Regenerative Rankine Cycle power plant.
Understanding the Regenerative Rankine Cycle efficiency formula
Moving on to a crucial aspect of any cycle – efficiency. The efficiency (\( η \)) of a Regenerative Rankine Cycle is a measure of how effective it converts input heat into output work. It is computed as the ratio of net work output to total heat input.
Let's denote the work done by the pump (\( W_{pump} \)) and the work done by the turbine (\( W_{turbine} \)). Then, we have the following formula for net work output (\( W_{net,out} \)):
\[ W_{net,out} = W_{turbine} - W_{pump} = m \cdot (h_{3} - h_{2}) - m \cdot (h_{5} - h_{4}) \]And this is how we denote the heat input, \( Q_{in} = m \cdot (h_{3} - h_{1}) \).
Therefore, we can define the efficiency formula, \( η_{th} = \frac{W_{net,out}}{Q_{in}} \), which gives the ratio of output work to input heat expressed as a percentage.
These formulas and computations allow you to better understand the energy transformations occurring in the cycle. An efficient Regenerative Rankine Cycle operation results in reduced fuel consumption and higher power output, bolstering the overall performance of power plants.
To conclude, the Regenerative Rankine Cycle initiatives a new approach to heat recovery and reuse systems. This cycle allows heat involved processes to be more economical and efficient, making this ideal for energy production and demanding industrial processes.
Studying Regenerative Rankine Cycle with Closed Feedwater Heater
When exploring the intricacies of the Regenerative Rankine Cycle, the role of the closed feedwater heater cannot be overstated. This central component not only enhances the working of the cycle but it significantly improves the thermal efficiency of the power plant as well. Its placement and function within the cycle bear direct implications on the overall energy transfer efficiency.
The Role of Closed Feedwater Heater in Regenerative Rankine Cycle
In the Regenerative Rankine Cycle, a heat exchange process operates between steam being expanded in the turbine and the feedwater pumped towards the boiler. Here, heat flows from high-temperature steam to low-temperature water. This heat transfer is facilitated through a device called the closed feedwater heater.
A closed feedwater heater is essentially a heat exchanger in which heat is transferred from the steam tapped from the turbine to the feedwater returning to the steam generator. It operates by directly mixing the feedwater with the extracted steam. This mixing process occurs in a closed environment, hence the nomenclature.
The mechanism of operation can be summarised as follows:
- The tapped steam, also known as bleed steam or extraction steam, enters the heater at high temperature.
- The feedwater, at a lower temperature, also enters the heater, though in a different section.
- Heat exchange occurs through the separation wall between the sections, with heat flowing from the steam to the feedwater.
- The warmed up feedwater is then sent back to the boiler, thus reducing the energy required by the boiler to transform it into steam.
The main objective of the closed feedwater heater in a Regenerative Rankine Cycle is to heat the feedwater to the maximum extent possible by the turbine extraction steam. Thereupon, it significantly reduces the fuel consumption of the entire cycle and improves the thermal efficiency of the power plant.
Enhancing Efficiency Through Regenerative Rankine Cycle with Closed Feedwater Heater
The Regenerative Rankine Cycle with a closed feedwater heater enables a substantial enhancement in the efficiency of power generation. As expressed earlier, it does so by effectively utilising the heat from the bled steam and transferring it to the feedwater.
One notable way it optimises efficiency is by reducing the demand for extra energy in heating the feedwater. The fact that the feedwater has already gained heat from the bled steam remarkably reduces the quantity of heat required by the boiler to convert the feedwater into steam.
Consequently, the cycle's overall work output enhances while the demands for energy input minimises. This leads to amplified thermal efficiency, calculated as the ratio of the work output to the heat input.
In a mathematical context, the efficiency (\( η_{th} \)) can be expressed as:
\[ η_{th} = \frac{W_{net,out}}{Q_{in}} \]Where:
- \( W_{net,out} \) stands for the net work output of the cycle, and
- \( Q_{in} \) signifies the total heat input to the cycle.
Higher the value of \( W_{net,out} \) and lower the value of \( Q_{in} \), greater will be the thermal efficiency. Implementing the Regenerative Rankine Cycle with a closed feedwater heater indeed increase \( W_{net,out} \) and decrease \( Q_{in} \), thus elevating \( η_{th} \).
Hence, the closed feedwater heater's operation within the Regenerative Rankine Cycle plays a pivotal role in improving the efficiency of power generation systems. By effectively harnessing the potential of the bleed steam, promoting a more sustainable use of resources, the integration of closed feedwater heaters establishes a significant forward leap in energy generation and conservation practices.
Regenerative Rankine Cycle - Key takeaways
- Regenerative Rankine Cycle comprises of four major components: boiler, turbine, condenser, and pump.
- A feedwater heater is a crucial component of the cycle that pre-warms the water using heat from the turbine exhaust steam to increase the overall efficiency.
- In a Regenerative Rankine Cycle, steam is bled off at various stages for feedwater heating, enhancing the plant's efficiency and conserving fuel.
- The thermal efficiency of Regenerative Rankine Cycle can be computed using the formula: ηth = Wnet,out/Qin, where Wnet,out is the net power output, and Qin is the heat input.
- All practical applications of the Regenerative Rankine Cycle revolve around efficient conversion of heat into work. It finds widespread use in power and energy production, industrial operations, and the marine industry.
- The formula basis of a Regenerative Rankine Cycle are based on heat and work transfer computations and efficiency analysis.
- Besides improving the thermal efficiency of power plants, the closed feedwater heater plays a crucial role in heat exchange processes in a Regenerative Rankine Cycle. It facilitates heat transfer from the high-temperature steam to the low-temperature water.
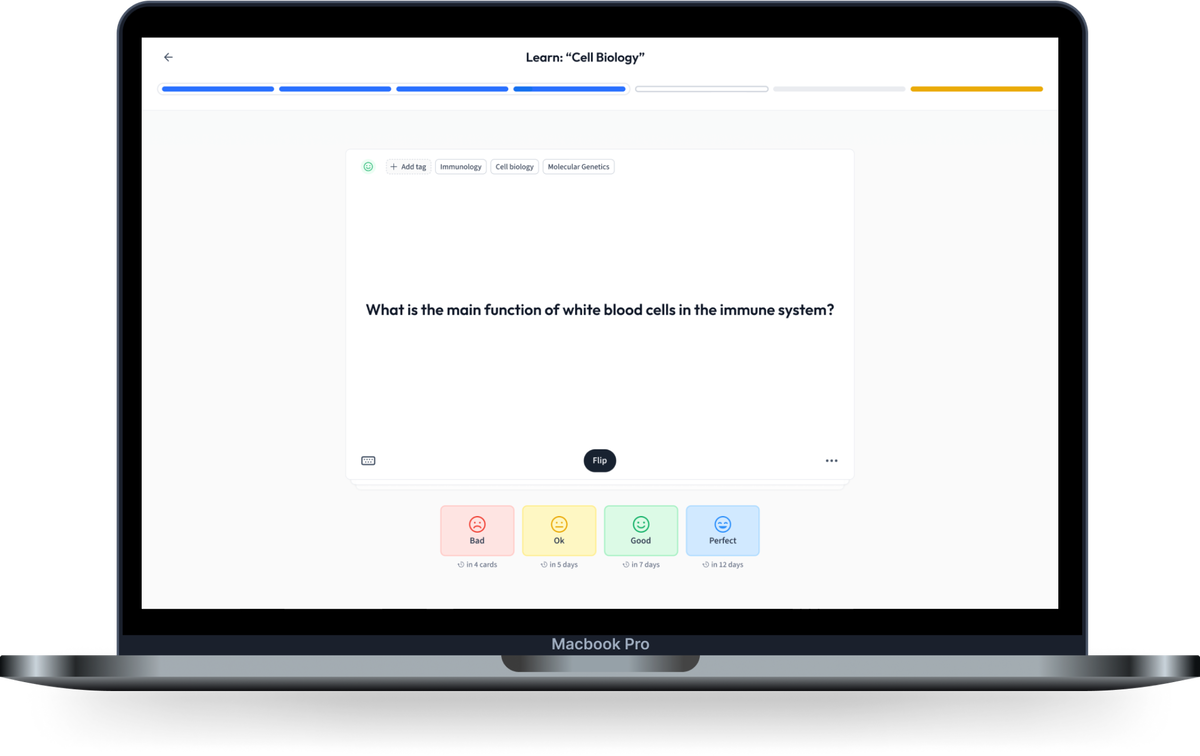
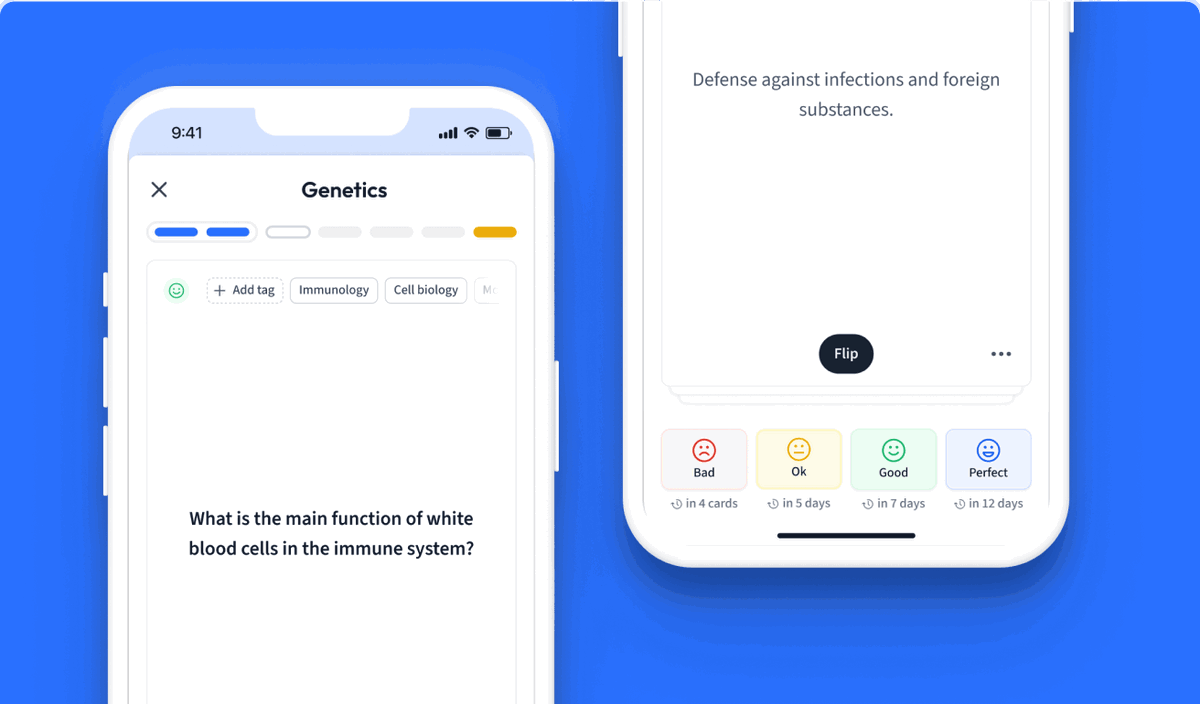
Learn with 30 Regenerative Rankine Cycle flashcards in the free StudySmarter app
We have 14,000 flashcards about Dynamic Landscapes.
Already have an account? Log in
Frequently Asked Questions about Regenerative Rankine Cycle
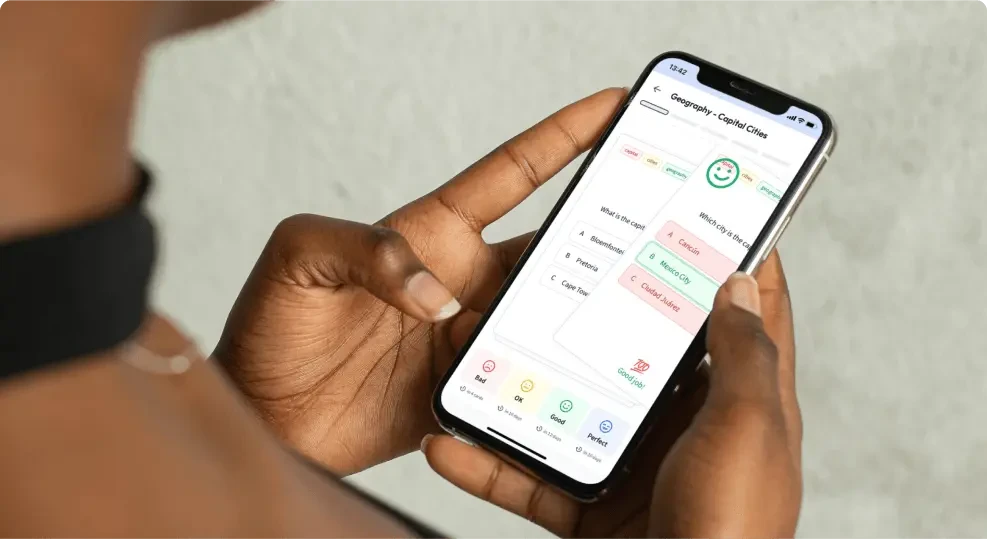
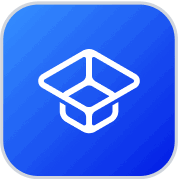
About StudySmarter
StudySmarter is a globally recognized educational technology company, offering a holistic learning platform designed for students of all ages and educational levels. Our platform provides learning support for a wide range of subjects, including STEM, Social Sciences, and Languages and also helps students to successfully master various tests and exams worldwide, such as GCSE, A Level, SAT, ACT, Abitur, and more. We offer an extensive library of learning materials, including interactive flashcards, comprehensive textbook solutions, and detailed explanations. The cutting-edge technology and tools we provide help students create their own learning materials. StudySmarter’s content is not only expert-verified but also regularly updated to ensure accuracy and relevance.
Learn more