Jump to a key chapter
Understanding Combustion Instability
Combustion instability is a critical factor to consider in the design and operation of engines, particularly in aerospace applications. It can affect the performance and safety of engines, making its study essential for engineers and scientists.
What is Combustion Instability?
Combustion instability refers to the undesirable oscillations in a combustion system caused by the interaction between the combustion process and the acoustic modes of the system.
This phenomenon occurs when the rate of heat released by the combustion interacts with the acoustic pressure waves within the combustion chamber, leading to oscillations. These oscillations can vary in magnitude, potentially causing damage to the combustion system if they become too severe.Understanding the mechanisms behind combustion instability helps in designing more efficient and safer engines and energy systems.
Example: One common example of combustion instability can be observed in rocket engines, where fluctuations in the combustion process can lead to highly destructive vibrations, endangering the mission and the vehicle itself.
Combustion Instability Causes
Several factors contribute to combustion instability, and pinpointing these causes is crucial for mitigating its effects. These factors can be broadly categorised into physical processes inside the combustion chamber and external influences.
Physical processes include:
- Fuel and air mixture anomalies
- Acoustic wave generation
- Heat release fluctuations
- Changes in operational conditions
- Component deterioration or failure
- System design flaws
Deep Dive: The Rayleigh criterion is a fundamental concept in understanding combustion instability. It states that instability occurs when pressure oscillations are in phase with heat release oscillations. Analysing this relationship helps in identifying and mitigating potential instability in combustion systems.
The Importance of Studying Combustion Instability
The study of combustion instability is of paramount importance for several reasons, primarily focusing on improving engine efficiency, reducing emissions, and ensuring safety. By understanding and addressing the causes of instability, engineers can design more reliable and effective combustion systems.Furthermore, with the push towards sustainable energy sources and the need for high-efficiency systems, reducing instability can make a significant contribution to achieving these goals. Effective management of combustion processes leads to better fuel utilisation and lower environmental impact.
Advancements in computational models and experimental techniques have greatly enhanced the ability to predict and mitigate combustion instability, leading to safer and more efficient engines.
Combustion Instability in Rocket Engines
Combustion instability in rocket engines poses significant challenges in aerospace engineering, affecting both the reliability and performance of these critical propulsion systems. Addressing this issue is paramount for ensuring the success of both manned and unmanned missions.
Combustion Instability in Liquid Rocket Engines
Liquid rocket engines, which use liquid fuel and oxidiser, are particularly susceptible to combustion instability. This problem arises from the complex interplay between the liquid propellant flow dynamics and the combustion process within the engine's combustion chamber.The nature of the liquid fuel and its interaction with the combustion process can introduce various oscillation modes, which are critical to understand and control for ensuring stable engine operation.
Example: A well-documented instance of combustion instability occurred in the development of the F-1 engines used in the Saturn V rocket. Engineers had to undertake extensive testing and design modifications to mitigate the oscillations observed during test firings.
Acoustic Coupling: A phenomena where the pressure waves within the engine's combustion chamber interact with the natural resonances of the system, leading to amplification of oscillations.
The process of mitigating combustion instability in liquid rocket engines often involves a combination of experimental testing and computational modelling. Techniques such as altering the geometry of the combustion chamber, introducing acoustic dampers, and modifying the injection system are common strategies employed by engineers to reduce the risks associated with these oscillations.
Combustion Instability in Solid Rocket Motors
Solid rocket motors, which utilise a solid propellant, also face challenges related to combustion instability. The nature of solid propellants means that once ignition occurs, the combustion process is less controllable compared to liquid rocket engines. Instabilities in solid rocket motors often arise from the interaction between the burning propellant surface and the acoustic modes of the combustion chamber.Understanding the characteristics of the solid propellant, including its burn rate and response to pressure oscillations, is crucial in minimising the risk of instability.
Erosive Burning: An escalated burn rate in specific regions of the solid propellant due to increased localised gas flow velocity, often contributing to combustion instability in solid rocket motors.
Example: Combustion instability in solid rocket motors was a critical issue during the development of the Space Shuttle's Solid Rocket Boosters (SRBs). Significant engineering efforts were focused on understanding and controlling the burn characteristics of the propellant to ensure stable operation throughout the flight.
Modern advancements in both liquid and solid rocket technology increasingly rely on sophisticated computational fluid dynamics (CFD) models to predict and mitigate combustion instability.
Advanced materials and manufacturing techniques have also played a crucial role in addressing combustion instability. In solid rocket motors, for example, the uniformity of the propellant's grain structure is paramount. Techniques such as additive manufacturing (3D printing) are being explored to produce more consistent and reliable propellant grains.
Combustion Instabilities in Gas Turbine Engines
Combustion instabilities in gas turbine engines present significant challenges in the field of aerospace and energy production. Addressing these instabilities is crucial for enhancing the performance, reliability, and safety of gas turbines.
Theoretical Analysis of Combustion Instability in Gas Turbines
To effectively mitigate combustion instabilities, one must first understand the underlying mechanisms that cause them. Theoretical analysis reveals that these instabilities are primarily the result of complex interactions between the engine's acoustic environment and the combustion process.Key concepts in this analysis include the Rayleigh criterion, which provides a condition under which combustion instabilities arise. According to this criterion, instabilities occur when there is a positive correlation between pressure fluctuations and heat release fluctuations within the combustion chamber.
Rayleigh criterion: A principle stating that combustion instability occurs when the product of pressure fluctuations and heat release fluctuations is positive, indicating that these two are in phase.
Example: In turbines, a combustor that is not properly designed may facilitate the interaction of flame dynamics with acoustic waves, resulting in a rise in instability as predicted by the Rayleigh criterion.
Exploring the various types of instabilities, such as thermoacoustic or acoustic coupling, reveals the multifaceted nature of this challenge. Thermoacoustic instabilities, for instance, involve the feedback loop between heat release from the combustion process and acoustic waves in the engine, amplifying the oscillations. Understanding these interactions on a theoretical level allows engineers to design components and control strategies that mitigate the instabilities before they translate into operational problems.
Mitigating Combustion Instabilities in Gas Turbine Engines
Mitigation of combustion instabilities involves a combination of design modifications, control strategies, and operational practices. Effective strategies often include:
- Optimisation of combustor geometry to disrupt harmful acoustic modes.
- Introduction of damping devices to absorb energy from acoustic waves.
- Adjustment of fuel injection systems to stabilise the combustion process.
Active control systems: Advanced technology that dynamically adjusts parameters such as fuel flow or air supply in response to real-time measurements, aiming to stabilise the combustion process and mitigate instabilities.
Example: One practical application of active control systems is the use of actuators to inject small amounts of air or fuel into the combustion chamber at precise moments, counteracting the development of instabilities.
Recent advancements in machine learning have led to the development of predictive models that can forecast the onset of combustion instabilities, allowing for preemptive adjustments.
Beyond traditional engineering solutions, leveraging computational fluid dynamics (CFD) for the design and optimisation of combustion systems plays a critical role. These powerful simulations can predict how changes in design or operation affect combustion dynamics, guiding the development of more stable engines. Furthermore, integration of CFD models with machine learning algorithms promises an even more sophisticated approach to diagnosing and counteracting instabilities in real time.
Combustion Instability Mitigation Techniques
Overview of Combustion Instability Mitigation Techniques
Mitigating combustion instability involves a comprehensive approach, encompassing both preventative measures in the design phase and corrective actions during operation. Techniques vary based on the specific type of engine or system, but they generally focus on disrupting the feedback loops that contribute to instability. Key Techniques include:
- Modifying combustor geometry to alter acoustic properties.
- Introducing damping devices to absorb undesirable oscillations.
- Optimising fuel injection patterns to stabilise combustion.
- Implementing active control systems that adapt to real-time combustion conditions.
Case Studies: Mitigating Combustion Instability in Aerospace Engineering
The aerospace industry has faced and overcome challenges related to combustion instability through a combination of experimental research and innovative engineering solutions. Selected case studies highlight the application and effectiveness of mitigation techniques in real-world scenarios.These case studies demonstrate not only the complexity of combustion instability issues but also the ingenuity and technological prowess applied to resolve them.
Deep Dive into Plasma Injection: In recent years, plasma injection technology has emerged as a novel approach to controlling combustion instability. By introducing plasma actuators into the combustion chamber, engineers can modify the flow and combustion characteristics almost instantaneously, offering a potential avenue for active control of instability. This technique has shown promise in experimental setups and could revolutionise stability management in aerospace engines.
Example: F-1 Engine Modifications: The development of the F-1 engines used in the Saturn V moon missions faced significant combustion instability challenges. Extensive experimental testing led to the introduction of baffles in the combustion chamber, effectively breaking up oscillation patterns and mitigating instability. This example underscores the importance of empirical testing and innovation in overcoming engineering hurdles.
The success of mitigation techniques often depends on a deep understanding of both the theoretical aspects of combustion dynamics and practical insights from experimental research.
Active Control Systems: These are sophisticated feedback systems that monitor engine performance indicators (such as pressure and temperature) in real-time and make adjustments to operational parameters to maintain stability within the combustion chamber. Their deployment in engines represents the cutting edge of mitigation technology.
Combustion Instability - Key takeaways
- Combustion Instability: Oscillations in a combustion system due to the interaction between combustion and the system's acoustic modes.
- Combustion Instability Causes: Fuel and air mixture anomalies, acoustic wave generation, heat release fluctuations, changes in operational conditions, component degradation, and design flaws.
- Rayleigh Criterion: A theoretical analysis tool stating instability occurs when pressure oscillations are in phase with heat release oscillations.
- Mitigation Techniques: Modifying combustor geometry, introducing damping devices, altering fuel injection, and implementing active control systems.
- Theoretical and Practical Analysis: Employing computational models and experimental techniques for predicting and mitigating combustion instability in various engines like liquid and solid rocket motors, and gas turbine engines.
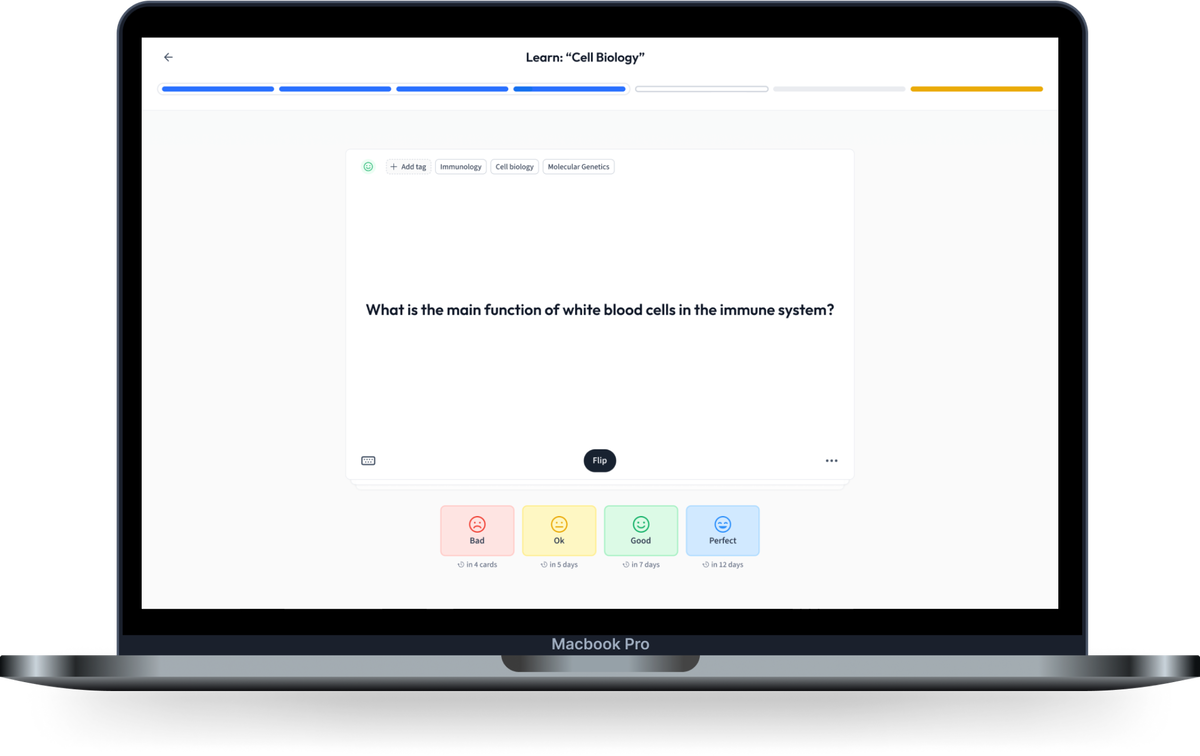
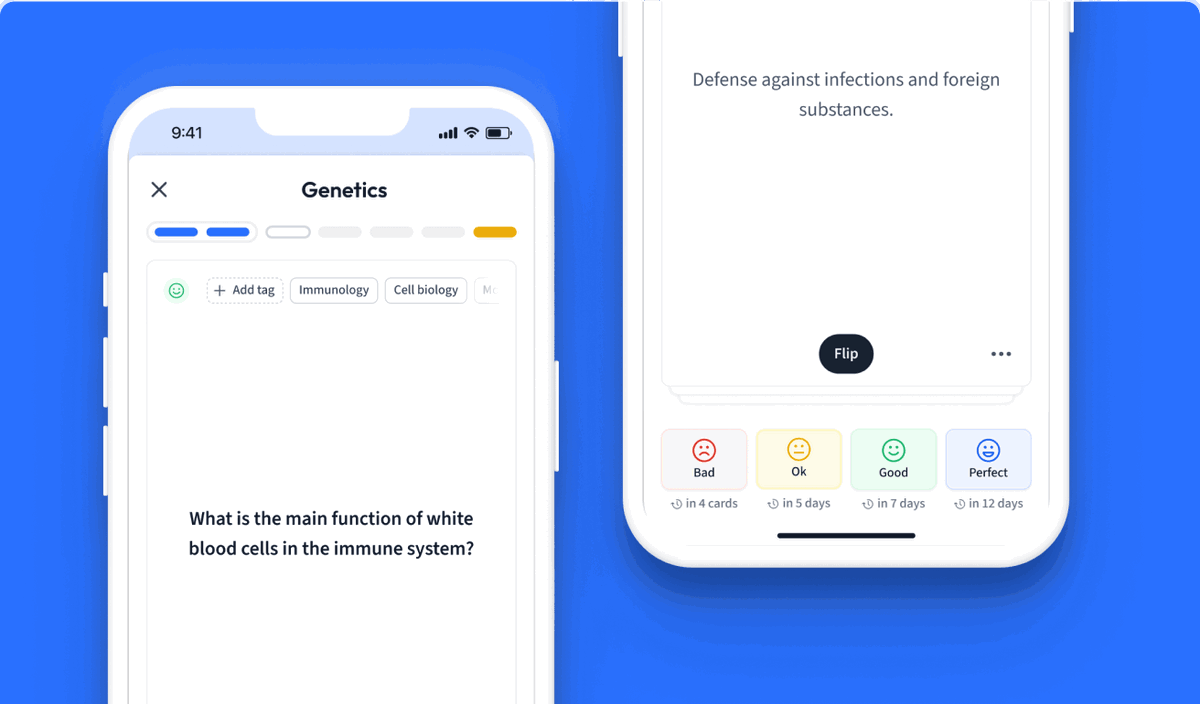
Learn with 12 Combustion Instability flashcards in the free StudySmarter app
We have 14,000 flashcards about Dynamic Landscapes.
Already have an account? Log in
Frequently Asked Questions about Combustion Instability
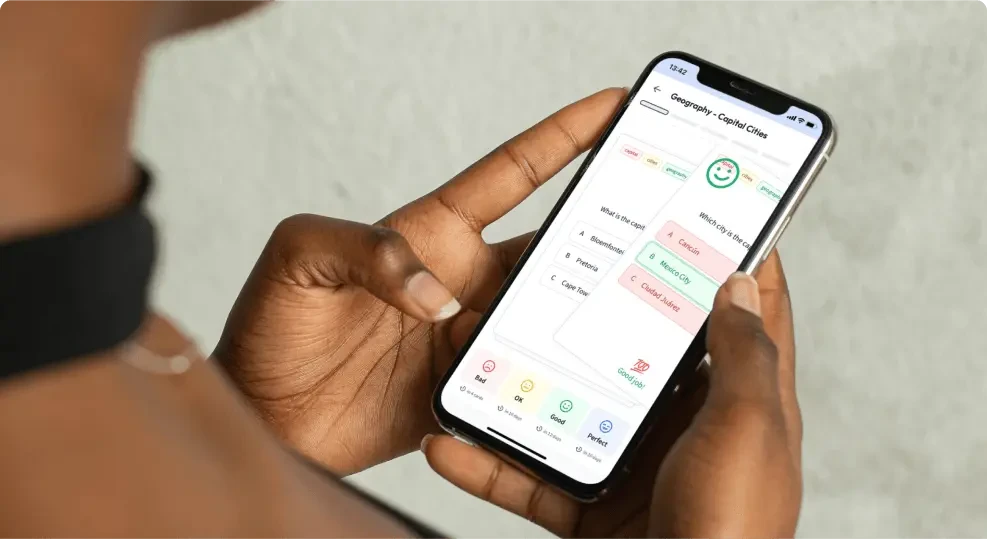
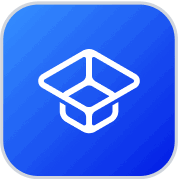
About StudySmarter
StudySmarter is a globally recognized educational technology company, offering a holistic learning platform designed for students of all ages and educational levels. Our platform provides learning support for a wide range of subjects, including STEM, Social Sciences, and Languages and also helps students to successfully master various tests and exams worldwide, such as GCSE, A Level, SAT, ACT, Abitur, and more. We offer an extensive library of learning materials, including interactive flashcards, comprehensive textbook solutions, and detailed explanations. The cutting-edge technology and tools we provide help students create their own learning materials. StudySmarter’s content is not only expert-verified but also regularly updated to ensure accuracy and relevance.
Learn more