Jump to a key chapter
Introduction to Liquid Rocket Engines
Liquid rocket engines represent the pinnacle of rocket technology, propelling spacecraft beyond the bounds of our planet. Understanding these sophisticated engines is essential for anyone fascinated by the vastness of space exploration.
What are Liquid Rocket Engines?
Liquid rocket engines are a type of rocket engine that uses liquid propellants for thrust. Unlike solid rocket engines that use a solid propellant, liquid engines have several advantages, including the ability to control and shut down the engine during flight, making them a preferred choice for manned missions.
Liquid Propellants: Substances in liquid form used as fuel and oxidiser to produce thrust in rocket engines. They are stored in separate tanks and mixed in the combustion chamber where they react and expand, providing propulsion.
The largest liquid-fuel rocket engine ever developed was the F-1, used in the Saturn V that sent Apollo astronauts to the Moon.
Fundamental Concepts of Liquid-Propellant Rocket Engines
To grasp the fundamentals of liquid rocket engines, it is crucial to understand the core concepts that govern their operation. These engines operate on Newton's third law of motion: for every action, there is an equal and opposite reaction. Combustion of liquid propellants generates hot gases that are expelled through a nozzle, propelling the rocket forward.
The working of liquid rocket engines involves various components and processes. The key components include the combustion chamber, where fuel and oxidiser mix and combust; the pumps, which feed the propellants into the combustion chamber; the nozzle, which accelerates the exhaust to produce thrust; and the thrust chamber, which directs the thrust for rocket propulsion. Some advanced engines also feature turbopumps to efficiently pump the fuels into the combustion chamber, and gimbal systems for steering the rocket.
An example of a liquid rocket engine is the Merlin engine developed by SpaceX, used in the Falcon 9 rocket. It uses liquid oxygen (LOX) as the oxidiser and RP-1, a refined form of kerosene, as the fuel. The Merlin engine showcases how advanced liquid-propellant technology can enable reusable rockets, significantly reducing the cost of space travel.
Liquid rocket engines allow for precise control over the vehicle's thrust and direction, making them ideal for inserting satellites into orbit and for manned spaceflights.
Thrust: The force generated by the rocket's engine to propel the rocket upwards against the force of gravity. It is a direct result of the combustion of propellants in the engine.
The History of Liquid Propellant Rocket Engines
The journey of liquid propellant rocket engines is a testament to human ingenuity and the relentless pursuit of exploration. From early experiments to powering spacecraft beyond the Earth's atmosphere, these engines have played a pivotal role in expanding our understanding of space.
Pioneering the Future: Early Innovations in Liquid Rocket Engines
The story of liquid rocket engines begins in the early 20th century, marked by the innovative experiments of rocketry pioneers. The development of the first liquid-fuel rocket by Robert H. Goddard in 1926 set the stage for future advancements in rocket technology. Goddard's pioneering work proved that liquid fuel could be more efficient than solid rockets, introducing a new era in space exploration. Following Goddard, pioneers across the world, including Hermann Oberth in Germany and Sergei Korolev in the Soviet Union, contributed significantly to the development and improvement of liquid rocket engines, paving the way for the use of these engines in ballistic missiles and space exploration vehicles.
An example of early innovation is the V-2 rocket developed by Wernher von Braun and his team during World War II. This ballistic missile, powered by a liquid propellant rocket engine using liquid oxygen and ethanol, demonstrated the potential for long-range warfare but also laid the groundwork for post-war space exploration efforts.
Many techniques and principles developed during the early days of liquid rocket engines are still in use today, showing the enduring legacy of the pioneers of rocketry.
Major Milestones in Liquid Propellant Rocket Development
Following the initial breakthroughs, several key milestones have marked the evolution of liquid propellant rocket engines. The transition from military to civilian applications, particularly in space exploration, saw liquid rockets becoming the backbone of modern space travel.
Among the major milestones:
- The successful launch of Sputnik in 1957 by the Soviet Union, the first artificial Earth satellite, powered by a liquid rocket engine.
- The development of the Saturn V rocket, which powered the Apollo moon landings, showcasing the capabilities of large-scale liquid engines.
- The Shuttle Main Engine (SSME), used on the Space Shuttle from the 1980s until 2011, demonstrated the reusability of liquid rocket engines.
- More recently, the development of the Raptor engine by SpaceX, designed for the Starship spacecraft, signifies the next generation of liquid rocket technology with its full-flow staged combustion cycle for improved efficiency and reusability.
The evolution of liquid rocket engine technology is marked by an ongoing quest for efficiency, reliability, and sustainability. Innovations such as the development of cryogenic fuel engines, which use super-cooled liquid hydrogen as fuel, have greatly increased the performance of rockets. Additionally, the move towards reusability, as seen with SpaceX's Falcon 9 rocket, aims to reduce the cost of access to space, making it more accessible for both commercial and scientific purposes. This shift not only represents a significant technological achievement but also a new economic model for space exploration, where the once prohibitive cost of rocket launches is drastically reduced.
The Space Shuttle's RS-25 engines were the first reusable liquid-fuel engines used for orbital missions, showcasing a significant milestone in the history of space exploration.
Design of Liquid Propellant Rocket Engines
Liquid propellant rocket engines are at the heart of today's space exploration and satellite deployment missions. Their design combines high-engineering precision with the fundamental laws of physics to achieve the necessary thrust and efficiency for space travel.
Core Design Principles of Liquid Rocket Engines
The core design principles of liquid propellant rocket engines focus on achieving maximum efficiency and reliability. These engines operate by propelling a spacecraft through the expulsion of mass at high speed, adhering to Newton's third law of motion. The liquid propellant, consisting of a fuel and an oxidiser, is carefully selected to ensure a controlled and powerful combustion process.
Efficiency in these engines is measured in terms of specific impulse, which represents the amount of thrust produced per unit of propellant. High specific impulse is desirable as it indicates a more efficient engine. The design also prioritises safety and controllability, incorporating mechanisms for throttling, shutdown, and restart during flight.
The combustion chamber and nozzle design are critical to a liquid rocket engine's performance. The chamber must withstand extreme temperatures and pressures, necessitating the use of advanced materials and cooling systems. The nozzle, often employing a bell-shaped design, is optimised for converting the high-pressure combustion gases into directed thrust. This involves complex fluid dynamics calculations to ensure that the flow remains smooth (laminar) and maximises velocity. Innovations in materials science and cooling techniques, such as regenerative cooling where the propellant is passed through channels in the engine wall before combustion, have significantly advanced the capabilities of these engines.
Innovations and Advances in Liquid Rocket Engine Design
Recent innovations and advances in liquid rocket engine design aim to improve efficiency, reduce costs, and enhance reusability. These developments cover every aspect of engine design, from the choice of propellants to the manufacturing processes used to create engine components.
One of the significant advances is the move towards full-flow staged combustion engines, which offer greater efficiency by pre-burning propellants in separate pre-combustion chambers before the main combustion chamber. This approach improves performance and reduces engine wear, contributing to the goals of reusability.
Full-Flow Staged Combustion: A combustion cycle in liquid rocket engines where both fuel and oxidiser are completely gasified in separate preburners before entering the main combustion chamber. This process maximises propellant combustion efficiency and engine performance.
The SpaceX Raptor engine embodies the cutting-edge design of modern liquid rocket engines. Utilising methane as fuel and oxygen as an oxidiser, it features full-flow staged combustion for enhanced efficiency. This design supports SpaceX's goal of creating a fully reusable spacecraft, significantly impacting cost and sustainability in space exploration.
Methane is becoming a preferred fuel in new rocket designs due to its high performance and the possibility of sourcing it from extraterrestrial bodies, supporting future long-duration space missions.
Additive manufacturing, or 3D printing, has revolutionised liquid rocket engine production. Components that once required extensive machining and assembly can now be printed in a single piece, reducing production time and cost while increasing structural integrity and design flexibility. This has not only accelerated the development of new engines but also allowed for bespoke designs that optimise performance and reduce weight.The use of advanced materials, such as superalloys capable of withstanding extreme temperatures and pressures without compromising their structural integrity, further enhances the capabilities of these engines. Such innovations underscore the evolving landscape of rocket engine design, driven by a quest for efficiency, reliability, and sustainability in space exploration.
Challenges in Liquid Rocket Engines
Liquid rocket engines, while essential for modern space exploration, face several significant challenges. Addressing these issues is crucial for improving engine reliability, efficiency, and safety. Two of the most pressing concerns include combustion instability and the development of effective cooling techniques.
Combustion Instability in Liquid Rocket Engines
Combustion instability in liquid rocket engines poses a major challenge, potentially leading to catastrophic engine failure. This phenomenon occurs when pressure waves within the combustion chamber amplify, causing oscillations that can damage engine components. Instability typically results from interactions between the flow of propellants, the geometry of the combustion chamber, and the combustion process itself.
Engineers combat this instability through careful design of the combustion chamber geometry, fine-tuning the mixture ratio of fuel and oxidiser, and employing active control techniques, such as acoustic dampers, to suppress the oscillations.
One notable instance of addressing combustion instability occurred during the development of the Saturn V rocket's F-1 engine. The engineering team implemented baffles inside the combustion chamber to destabilise the pressure waves, successfully mitigating the issue and ensuring the engine's reliability during the Apollo missions.
Combustion Instability: A condition in liquid rocket engines where fluctuations in the combustion process induce potentially damaging oscillations within the combustion chamber. It is a critical issue that can lead to engine failure if not properly managed.
Cooling Techniques in Liquid Rocket Engines
Maintaining operational temperatures within safe limits is another critical challenge for liquid rocket engines. The immense heat generated during combustion must be managed to prevent damage to the engine components. Effective cooling techniques are therefore integral to the design and operation of these engines.
Cooling methods include regenerative cooling, where some of the propellant is circulated around the combustion chamber and nozzle before being injected into the combustion chamber. This not only cools the engine parts but also pre-heats the propellant, improving overall engine efficiency.
Advanced cooling techniques also explore the use of exotic materials with higher melting points and better thermal conductivity, alongside innovative designs that maximise heat dissipation. These developments have led to significant improvements in engine durability and performance, enabling longer missions and the use of more powerful engines.
The Space Shuttle Main Engine (SSME) is an example of an engine that utilised sophisticated cooling techniques. It employed a complex network of tubes that carried liquid hydrogen around the combustion chamber and nozzle, effectively removing heat and preventing thermal damage under the extreme conditions of space flight.
Film cooling, another effective method, involves injecting a thin layer of coolant fluid that evaporates and forms a protective barrier on the hot engine surfaces, further enhancing thermal management.
Liquid Rocket Engines - Key takeaways
- Liquid Rocket Engines use liquid propellants and offer the ability to control and shut down the engine during flight, making them ideal for manned missions and precise spacecraft positioning.
- Fundamental concepts of Liquid-Propellant Rocket Engines include Newton's third law of motion for propulsion and essential components like the combustion chamber, pumps, nozzle, and thrust chamber.
- History of Liquid Propellant Rocket Engines dates back to Robert H. Goddard's first liquid-fuel rocket in 1926, leading to significant developments like the Saturn V rocket's F-1 engine and SpaceX's Raptor engine.
- Design principles of Liquid Propellant Rocket Engines focus on efficiency, specific impulse, and advanced cooling methods like regenerative cooling to manage the extreme heat generated during combustion.
- Challenges in Liquid Rocket Engines involve managing combustion instability and developing effective cooling techniques to ensure engine reliability and performance.
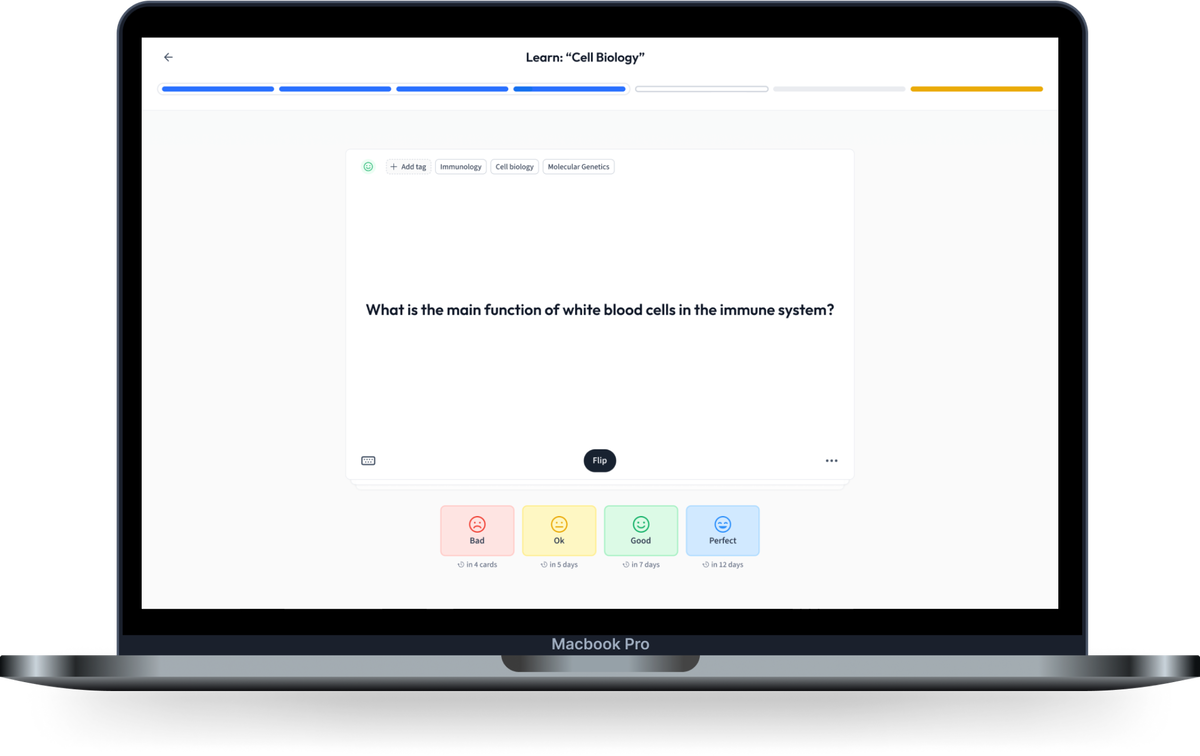
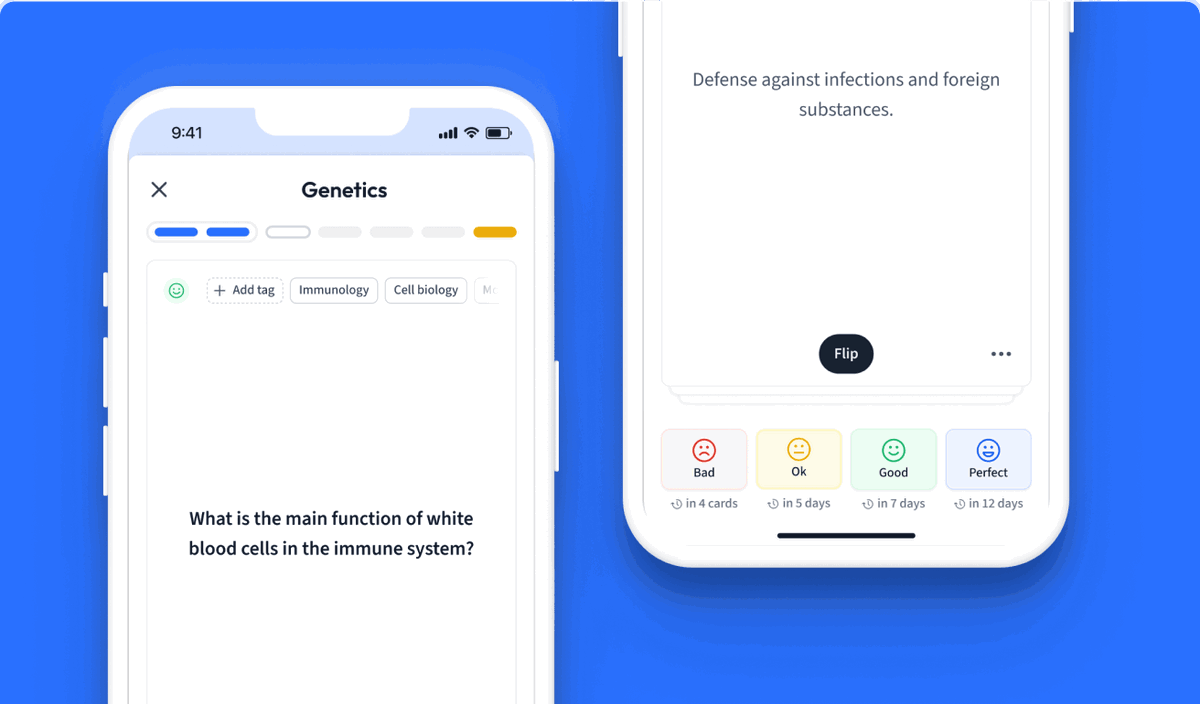
Learn with 12 Liquid Rocket Engines flashcards in the free StudySmarter app
We have 14,000 flashcards about Dynamic Landscapes.
Already have an account? Log in
Frequently Asked Questions about Liquid Rocket Engines
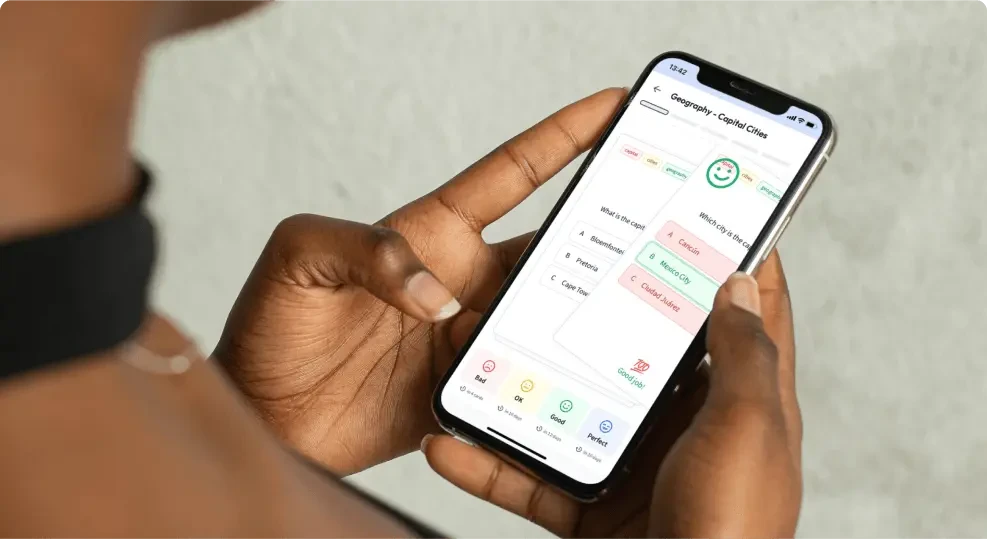
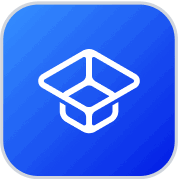
About StudySmarter
StudySmarter is a globally recognized educational technology company, offering a holistic learning platform designed for students of all ages and educational levels. Our platform provides learning support for a wide range of subjects, including STEM, Social Sciences, and Languages and also helps students to successfully master various tests and exams worldwide, such as GCSE, A Level, SAT, ACT, Abitur, and more. We offer an extensive library of learning materials, including interactive flashcards, comprehensive textbook solutions, and detailed explanations. The cutting-edge technology and tools we provide help students create their own learning materials. StudySmarter’s content is not only expert-verified but also regularly updated to ensure accuracy and relevance.
Learn more