Jump to a key chapter
What is Fatigue Analysis?
Fatigue analysis is a branch of engineering that studies the process through which materials deteriorate under the influence of cyclic loading over time. It is paramount in predicting the lifespan of materials and structures, particularly in contexts where safety and sustainability are crucial. The occurrence of fatigue is not instantaneous; rather, it develops as small cracks that grow incrementally, leading to failure.
Understanding Fatigue Analysis Definition
Fatigue analysis involves using various methods and principles to predict and evaluate the durability of materials or structures under repeated stress cycles. It bridges the gap between material science and structural engineering, offering insights into how and when materials might fail. Fatigue failure is distinct from other types of structural failure as it occurs under stress levels lower than the material's ultimate tensile strength.
Fatigue Analysis: A methodological approach to determine the fatigue life of a material or component under cyclic loading, which can include theoretical, experimental, and numerical techniques.
Example: A bridge exposed to thousands of vehicles passing daily undergoes fatigue analysis to predict when critical components might fail due to the constant stress, ensuring timely maintenance or component replacement.
The Importance of Fatigue Analysis in Aerospace Engineering
In aerospace engineering, fatigue analysis takes on a critical role due to the unique stresses aircraft components undergo. Aircraft are subject to extreme conditions varying from high-altitude thin air to the pressurised environment of cabins, alongside the cyclic stress of take-offs, landings, and turbulence. Fatigue analysis in this context ensures that aircraft can withstand these conditions over time without catastrophic failure, guaranteeing safety and reliability.
Deep Dive: The Comet, the world's first commercial jetliner, suffered a series of catastrophic failures in the early 1950s. Investigation revealed that these were caused by metal fatigue in the airframe, exacerbated by square windows which concentrated stress at the corners. This tragic example underscores the importance of fatigue analysis in design choices, leading to the rounded windows seen in today's aircraft.
The methods used in fatigue analysis, such as crack propagation models and S-N curves (stress-number of cycles), are fundamental in designing safe and durable aerospace components.
Common Causes of Fatigue Failure in Engineering
Fatigue failure in engineering manifests when a material or structural component fails to withstand the repeated application of loads below its ultimate tensile strength. This phenomenon often culminates in structural damage and, potentially, catastrophic failures. Identifying the causes of fatigue failure is pivotal in prolonging the lifespan of engineered systems and ensuring their reliability and safety. Several factors contribute to the onset of fatigue failure, ranging from material defects to environmental conditions, underscoring the multifaceted nature of this challenge.
Fatigue failure typically occurs in three stages: crack initiation, crack propagation, and ultimately, sudden fracture of the component.
Stress Concentration: Areas in a material or component that experience stress levels significantly higher than the surrounding region due to geometric features, such as holes, notches, or sharp corners, leading to an increased likelihood of fatigue failure.
Example: The presence of a small notch on a rotating shaft in machinery can act as a focal point for stress concentration, initiating the fatigue process and ultimately leading to failure.
Common causes of fatigue failure include:
- Material defects, such as inclusions or voids within the material, which act as crack initiators.
- Improper material selection or processing, leading to reduced resistance to cyclic loading.
- Design issues, such as inadequate consideration for load paths and stress concentration areas.
- Environmental factors, such as corrosion, which can exacerbate the development of fatigue cracks.
- Operational errors, including overload conditions or incorrect assembly, affecting the structural integrity.
Real-World Fatigue Analysis Example in Aerospace Components
The field of aerospace engineering provides a prime example of how critical fatigue analysis is in safeguarding against mechanical failure and ensuring operational safety. Aircraft components are routinely exposed to cyclic stressors that can initiate and propagate cracks, making fatigue analysis a non-negotiable aspect of their design and maintenance strategies. One key area where this analysis is particularly vital is in the examination of aircraft engines and fuselage structures. Here, the implications of fatigue failure are profound, with the potential to compromise the safety of flights and the lives of passengers.
Deep Dive: One of the most documented instances of fatigue failure in aerospace history involved the Aloha Airlines Flight 243 incident in 1988. A significant portion of the aircraft's upper fuselage tore away during flight due to widespread fatigue damage. This event was a stark reminder of the potential consequences of neglecting fatigue analysis in aircraft maintenance. The investigation spurred enhanced regulations on ageing aircraft, focusing on improved fatigue inspection and maintenance procedures.
Advanced techniques in fatigue analysis for aerospace components include the use of fracture mechanics to predict crack growth and non-destructive testing methods to detect early signs of fatigue damage.
Fatigue Analysis Techniques
Fatigue analysis is an essential task in ensuring the durability and reliability of materials and structures exposed to cyclic loading. Modern techniques in fatigue analysis blend experimental data with sophisticated computational models to predict how long materials can last under varying stress conditions before failure occurs. This predictive capability is paramount across many industries, including aerospace, automotive, and civil engineering, where safety and longevity are critical.
Overview of Modern Fatigue Analysis Techniques
The methods employed in modern fatigue analysis are diverse, each suited to different scenarios and types of materials. These techniques include but are not limited to, stress-life ( extbf{S-N}) curve methods, strain-life ( extbf{ε-N}) approaches, and fracture mechanics. Additionally, advancements in technology have paved the way for using finite element methods (FEM) for intricate and precise simulations.
- Stress-Life (S-N): Predominantly used for high-cycle fatigue analysis, where the number of cycles to failure is relatively high.
- Strain-Life (ε-N): Appropriate for low-cycle fatigue, involving the assessment of plastic strain.
- Fracture Mechanics: Focuses on the growth of a pre-existing flaw or crack under cyclic loading.
- Finite Element Methods (FEM): Used to simulate and analyse complex structures under real-world conditions, identifying critical stress points.
Modern fatigue analysis often utilises digital twins to simulate real-world conditions and predictive maintenance, greatly enhancing the accuracy of fatigue life predictions.
Fatigue Analysis Equations: A Deep Dive
Understanding the mathematical basis of fatigue analysis is crucial for accurately predicting material failure. The core of many modern analytical techniques is the application of fundamental equations and models that describe the behaviour of materials under cyclic loads. The primary equations in fatigue analysis include the Basquin equation for the stress-life method, \[ \sigma = \sigma_f' (2N_f)^b \], and the Coffin-Manson equation for the strain-life method, \[ \epsilon = \epsilon_f' (2N_f)^c + \sigma_f' (2N_f)^b/E \. Here:
- \( \sigma \) is the stress amplitude,
- \( \sigma_f' \) is the fatigue strength coefficient,
- \( N_f \) is the number of cycles to failure,
- \( b \) and \( c \) are the Basquin and Coffin-Manson exponents, respectively,
- \( E \) is the modulus of elasticity, and
- \( \epsilon_f' \) is the fatigue ductility coefficient.
The application of these equations extends beyond mere predictions of failure time. By incorporating them into computational models, engineers can simulate various loading conditions, material types, and geometrical configurations. This capability allows for the optimisation of designs and the identification of potential failure points before a prototype has even been created. Moreover, the ongoing development in materials science, especially with new alloys and composites, necessitates continuous updates to these models to accurately reflect material behaviour under cyclic loads. The collaboration between computational tools and experimental data is therefore a critical aspect of modern fatigue analysis, ensuring that predictions remain as accurate and relevant as possible.
Composite Fatigue Analysis
Composite fatigue analysis represents a specialised branch within materials engineering that addresses how composite materials behave under cyclic loading over time. Notably used in sectors like aerospace, automotive, and wind energy, composite materials offer superior strength-to-weight ratios but present unique challenges in fatigue assessment compared to traditional metals. This analysis helps predict the point at which these materials may start to exhibit signs of degradation or failure, which is crucial for designing safe and durable components.
Fundamentals of Composite Fatigue Analysis
Understanding the fundamentals of composite fatigue analysis involves recognising the distinctive nature of composite materials. These materials are made from two or more constituent materials with significantly different physical or chemical properties. When combined, they produce a material with characteristics different from the individual components. The cornerstone of composite fatigue analysis is the ability to predict the endurance of composite structures under repeated loads. This prediction is not straightforward due to the intricacies of composite material behaviour, including their anisotropic (directionally dependent) properties, and the variety of failure modes they exhibit.
Anisotropy: A material characteristic in which properties like strength and stiffness vary depending on the direction of the load. This trait is particularly pronounced in composite materials.
Example: In aerospace engineering, the wings of an aircraft made from carbon-fibre reinforced polymers must be analyzed for fatigue to ensure they can withstand varying loads during operations without failure. This includes taking into account the directional properties of the composite material for accurate analysis.
Fatigue in composites often starts at points of stress concentration such as holes or notches, or at interfaces between the different materials constituting the composite.
Challenges and Solutions in Composite Fatigue Analysis
Conducting fatigue analysis in composite materials introduces several challenges not present with homogeneous materials. These challenges stem from the complex microstructure of composites, including fibre-matrix interfaces, variation in fibre orientation, and the presence of voids. Solutions to these challenges often involve advanced modelling techniques, enhanced material characterisation methods, and development of more accurate predictive tools. Here are key challenges and their respective solutions:
- Variability in Material Properties: The anisotropic nature of composites can lead to significant variability in predicted versus actual performance. Solution: High-fidelity computational models, combined with extensive material testing, improve the accuracy of predictions.
- Detection of Early-stage Damage: Traditional non-destructive evaluation methods may not detect sub-surface cracks or delamination in composites. Solution: Advanced techniques such as ultrasonic phased array and thermography provide better detection capabilities.
- Modelling Complex Behaviour: The behaviour of composites under load is influenced by numerous factors, making modelling a challenge. Solution: Multi-scale modelling approaches, which consider everything from the microstructural to the structural level, offer more comprehensive analyses.
Deep Dive: One state-of-the-art solution in combating the variability in composite material properties involves the use of digital twins. A digital twin is a virtual replica of a physical product that can be used for in-depth analysis and simulation. By applying real-time data and advanced simulation techniques, engineers can predict fatigue life with greater accuracy and adjust designs preemptively to mitigate potential failure modes. The implementation of machine learning algorithms with digital twins represents a cutting-edge frontier in composite fatigue analysis, allowing for dynamic adjustments based on predictive models and real-world performance data.
Fatigue Analysis - Key takeaways
- Fatigue Analysis Definition: A branch of engineering studying material deterioration under cyclic loads to predict structural lifespan, distinguishing it from other failures by crack initiation and growth.
- Fatigue Failure Analysis: Investigates the causes and progression of fatigue in materials, which is crucial for enhancing the safety and longevity of engineering systems.
- Fatigue Analysis Equations: Includes key equations such as the Basquin equation for stress-life and the Coffin-Manson equation for strain-life, which predict material behaviour under cyclic loading.
- Fatigue Analysis Techniques: Encompass experimental, numerical, and theoretical methods like S-N curves, finite element methods (FEM), and fracture mechanics, to assess material durability.
- Composite Fatigue Player: Specialised analysis addressing the unique behaviour of composite materials under cyclic loading, including the prediction of different failure modes and the effects of anisotropy.
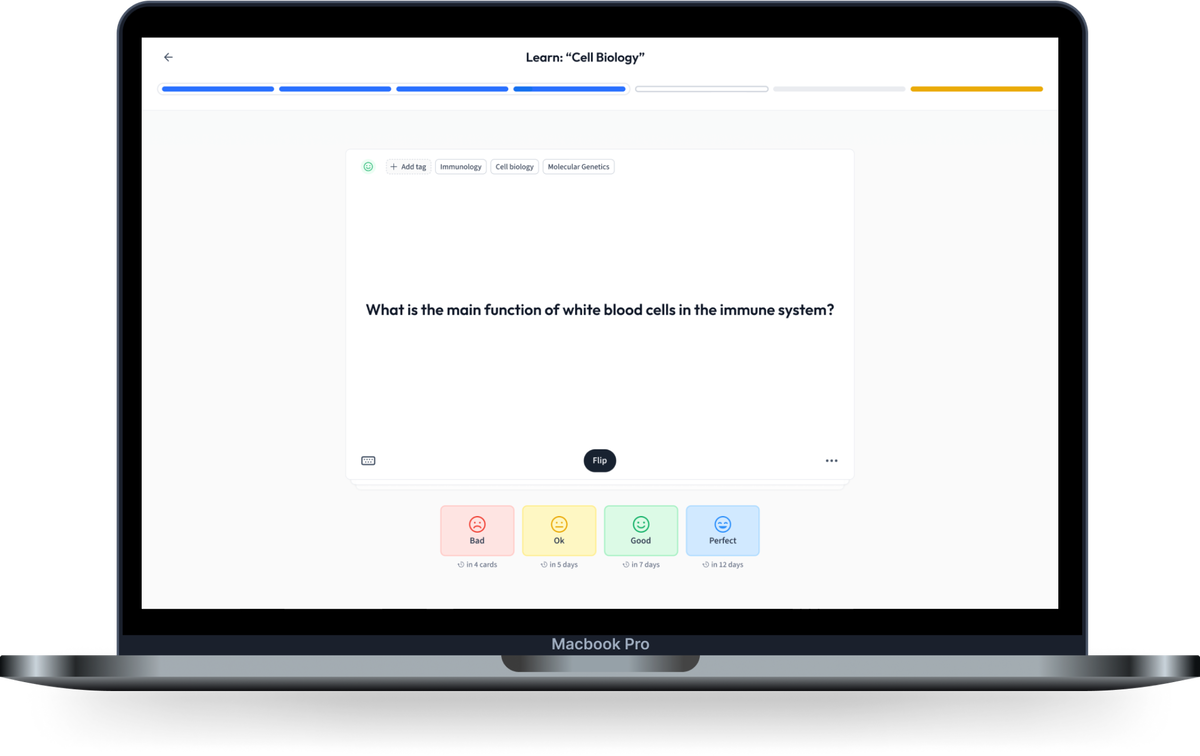
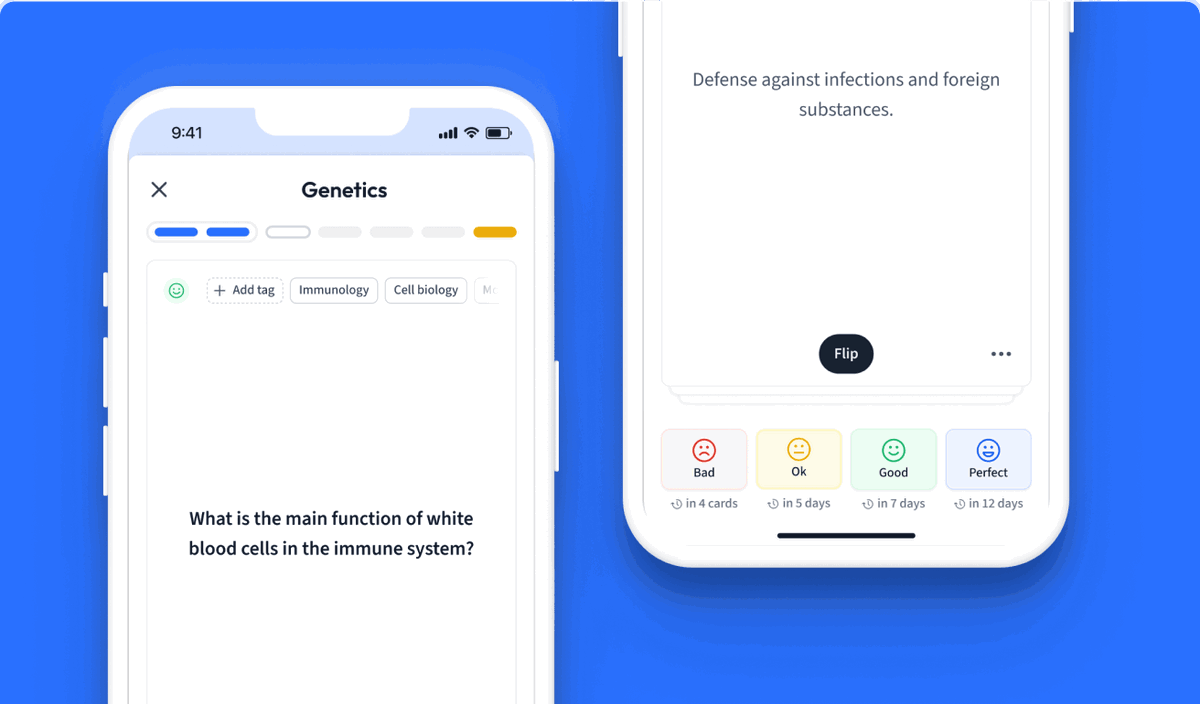
Learn with 12 Fatigue Analysis flashcards in the free StudySmarter app
We have 14,000 flashcards about Dynamic Landscapes.
Already have an account? Log in
Frequently Asked Questions about Fatigue Analysis
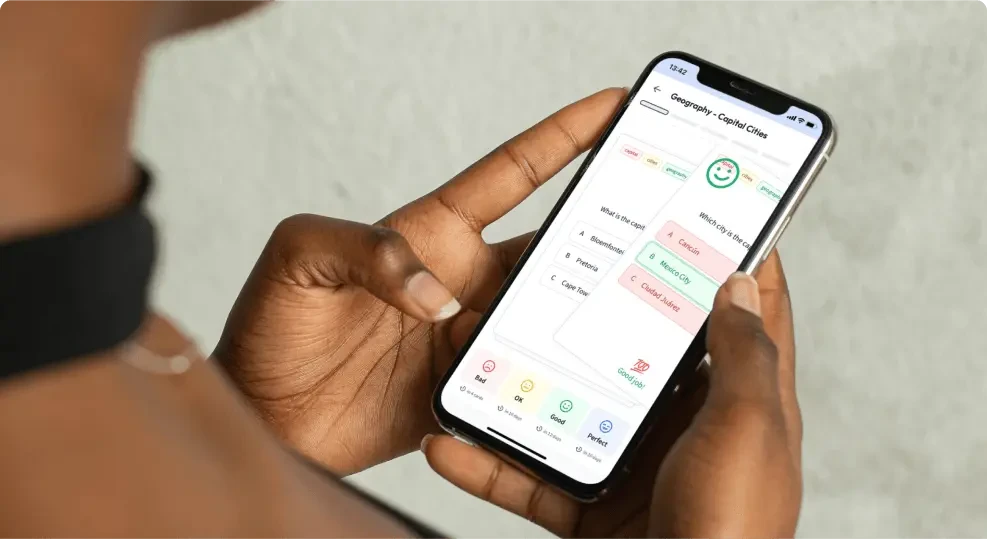
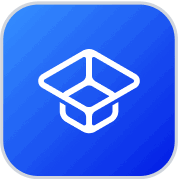
About StudySmarter
StudySmarter is a globally recognized educational technology company, offering a holistic learning platform designed for students of all ages and educational levels. Our platform provides learning support for a wide range of subjects, including STEM, Social Sciences, and Languages and also helps students to successfully master various tests and exams worldwide, such as GCSE, A Level, SAT, ACT, Abitur, and more. We offer an extensive library of learning materials, including interactive flashcards, comprehensive textbook solutions, and detailed explanations. The cutting-edge technology and tools we provide help students create their own learning materials. StudySmarter’s content is not only expert-verified but also regularly updated to ensure accuracy and relevance.
Learn more