A chemical equation is a symbolic representation of a chemical reaction where reactants are transformed into products. It showcases the substances involved and their quantitative relationships. Balancing chemical equations is crucial, as it ensures the conservation of mass and complies with the law of conservation of matter. Some common types of chemical equations include synthesis, decomposition, single replacement, and double replacement reactions. To fully understand a chemical reaction, one must learn to decode the chemical formulas and symbols that represent physical substances and their interactions.
Understanding Chemical Equations in Physical Chemistry
Chemical equations are fundamental to the study of physical chemistry, providing critical information about how substances interact on a molecular level.
What is a Chemical Equation: The Basics
A chemical equation is a written shorthand used by scientists to easily communicate complex chemical reactions. At its core, it represents the conversion of reactants (substances present before the reaction) into products (substances formed as a result of the reaction). Chemical equations are balanced to reflect the law of conservation of mass, meaning the number of atoms of each element is the same on both sides of the equation.
Chemical Equation: A representation of a chemical reaction that uses symbols and formulas to depict the substances involved, their relative amounts, and the direction of the reaction.
An example of a chemical equation is the reaction of hydrogen with oxygen to form water:
2H2 + O2 ightarrow 2H2O
The Role of Chemical Equations in Physical Chemistry
Within physical chemistry, chemical equations serve as the foundation for understanding how reactions occur and predicting the outcomes of such reactions. They are not only crucial for academic study but are also essential in practical applications like pharmaceuticals, industrial processes, and environmental science. By quantifying the relationships in a chemical reaction, chemical equations allow chemists to calculate yields, determine reaction rates, and establish the energy changes involved.
Chemical equations also underpin much of the work in thermodynamics and kinetics, two key disciplines within physical chemistry which dictate how reactions proceed and at what rates. For instance, the ability to read and understand a chemical equation is vital for calculating the Gibb's free energy, which predicts whether a reaction is spontaneous or non-spontaneous.
Chemical Equations Symbols and Their Significance
Chemical equations are comprised of symbols that convey specific information about the reaction. These include the chemical symbols for elements, such as 'H' for hydrogen and 'O' for oxygen, as well as formulas representing compounds. Additional symbols show the state of matter of the reactants and products, energy changes, and the conditions under which the reaction takes place.
State Symbols: Used in chemical equations to indicate the physical state of each substance. The common state symbols are:
- (s) for solids
- (l) for liquids
- (g) for gases
- (aq) for aqueous solutions, which are substances dissolved in water
Consider the equation for the combustion of methane:
CH4(g) + 2O2(g) ightarrow CO2(g) + 2H2O(l)
This equation indicates that methane and oxygen gases react to produce carbon dioxide gas and liquid water.
Interpreting Symbols for Better Comprehension
The accurate interpretation of symbols in chemical equations is key to understanding the specifics of a reaction. Each symbol and its placement convey vital details about the quantitative and qualitative aspects of the reaction. For instance, coefficients before a chemical formula represent the number of moles or molecules involved, while subscripts within a chemical formula show the number of atoms of each element in a molecule. Learning to read these symbols correctly can unveil the narrative of the chemical reaction, and allow the prediction of reaction behavior under different conditions.
Did you know the '+' symbol in a chemical equation suggests a combination of reactants, while the arrow ightarrow denotes the direction of the reaction from reactants to products?
Other notations such as catalysts, heat, and pressure may be indicated above or below the reaction arrow to provide context on the reaction conditions. For example,
2H2O2(aq) ightarrow 2H2O(l) + O2(g) catalyst: MnO2informs us that the decomposition of hydrogen peroxide is facilitated by the catalyst manganese dioxide (MnO2).
Mastering Balancing Chemical Equations
Balancing chemical equations is not only a skill crucial for chemistry students but also for professional chemists and scientists in various fields. It involves the detailed verification that the number of atoms for each element is the same on both sides of the equation, respecting the law of conservation of mass. Through balancing equations, one comprehends the stoichiometry of the reaction, allowing for accurate predictions and measurements in chemical processes.
Steps to Balance Chemical Equations
Balancing a chemical equation may seem daunting at first, yet by following systematic steps, one can master it with practice. Begin with identifying the reactants and products and write their correct formulas. Then, make a list of the number of atoms of each element present in both the reactants and products. Here are the sequential steps to walk through when balancing equations:
- Start with compounds that contain the rarest element.
- Balance elements that appear only in one reactant and one product, focusing on their coefficients.
- Balance hydrogen and oxygen atoms last as they are often present in multiple reactants and products.
- Use fractional coefficients if necessary and then multiply through by the smallest common multiple to convert all coefficients into whole numbers.
- Check your work to ensure that the number of atoms of each element matched on both sides of the equation.
It is essential to understand that the coefficients represent the molar proportions in the balanced equation, which can later be used to calculate the mass and volumes of reactants and products.
Consider the reaction of nitrogen gas reacting with hydrogen gas to form ammonia:
N2(g) + H2(g) ightarrow NH3(g)
This unbalanced equation does not respect the law of conservation of mass as the number of hydrogen atoms does not match on either side. The balanced equation looks like this:
N2(g) + 3H2(g) ightarrow 2NH3(g)
Remember to only adjust the coefficients when balancing chemical equations, never change the subscripts within the chemical formulas, as that represents a change in the actual compounds, not the amounts.
Common Challenges in Balancing Equations and Solutions
While balancing chemical equations is straightforward in theory, many students encounter challenges along the way. Some common issues include balancing equations with polyatomic ions, reactions that include complex molecules, and combustion reactions. A helpful approach is to consider polyatomic ions as single units if they appear unchanged on both sides of the reaction. For more complex molecules, break down the problem by balancing atoms of elements that are not found in other compounds first. Lastly, with combustion reactions, start with balancing the carbon and hydrogen atoms before oxygen, as these are typically present in excess.
It is also critical to be meticulous with bookkeeping, ensuring accurate tallies of each atom on both sides of the equation. Using a table to keep track of atoms or a step-by-step checklist can prevent confusion and mistakes.
Element | Reactants | Products |
Carbon (C) | 1 | 1 |
Hydrogen (H) | 4 | 4 |
Oxygen (O) | 2 | 2 |
This is an example of a simple table to tally the number of atoms of each element for the combustion of methane: CH4 + 2O2 ightarrow CO2 + 2H2O.
In some cases, unanticipated challenges arise, such as balancing redox reactions which involve a change in oxidation states. This typically requires a more complex method involving half-reactions where separate equations are written for the oxidation and reduction processes. These are then balanced individually for mass and charge before combining them to give a balanced overall equation.
For example, in the redox reaction where manganese dioxide reacts with hydrogen peroxide:
MnO2(s) + H2O2(l) ightarrow Mn2+(aq) + O2(g) + H2O(l)
This reaction is balanced by considering the half-reactions for the reduction of manganese and the oxidation of hydrogen peroxide, then balancing electrons to ensure charge conservation.
A chemical equation is a concise representation of a chemical reaction, indicating how reactants are converted into products. Balancing chemical equations is essential for ensuring that the equation obeys the law of conservation of mass by having an equal number of atoms for each element on both sides. Different types of chemical equations include synthesis, decomposition, and displacement reactions, each characterised by specific patterns of reactants and products. These equations are constructed using chemical formulas representing the substances involved, along with symbols that denote their states and the reaction's direction.
Exploring Types of Chemical Equations
Chemical equations offer more than just a snapshot of a reaction; they provide a window into the process of conversion from reactants to products. By analysing different types of chemical equations, one can gain insights into the nature of chemical reactions, understanding fundamental concepts of synthesis, decomposition, and displacement. Engaging with these various forms of chemical equations allows an appreciation for the complexity and diversity of chemical interactions.
What Constitutes a Synthesis Reaction?
Synthesis reactions, commonly known as combination reactions, are a type of chemical reaction where two or more simple substances combine to form a more complex product. They are among the most basic types of reactions studied in chemistry and serve as the foundational processes in the synthesis of countless compounds used in industries and laboratories. In synthesis reactions, there is typically a direct combination of elements or simpler compounds under certain conditions such as heat, pressure, or in the presence of catalysts.
The general formula for a synthesis reaction is:
A + B ightarrow AB
where A and B represent the reactants that combine to form the product AB. This form of reaction is fundamental to understanding how complex molecules are constructed from their elemental or simpler forms. It's reflective of numerous natural processes and widely applied in synthesising materials such as polymers, pharmaceuticals, and inorganic compounds.
Synthesis Reaction: A chemical reaction in which two or more reactants combine to form a single, more complex product.
An example of a synthesis reaction is the formation of water from hydrogen and oxygen gases:
2H2(g) + O2(g) ightarrow 2H2O(l)
In a synthesis reaction, energy is often released in the form of heat or light, making many of these reactions exothermic.
Synthesis reactions are not only simple combinations; they can also involve complex mechanisms in cases like the fixation of nitrogen from the atmosphere to form ammonia, a process essential for producing fertilisers. This particular synthesis reaction, known as the Haber process, occurs under high pressure and temperature and requires a catalyst to facilitate the combination of nitrogen and hydrogen molecules.
Decomposition Reactions: A Closer Look
Opposite to synthesis reactions are decomposition reactions, where a single compound breaks down into simpler substances or elements. This type of reaction often requires energy input in the form of heat, light, or electricity to overcome the chemical bonds holding the compound together. Notable for their role in processes such as electrolysis and the breakdown of organic matter, decomposition reactions are critical to understanding the degradation of compounds and recycling of elements in natural systems.
The general form of a decomposition reaction is as follows:
AB ightarrow A + B
where AB represents the complex compound that decomposes into its constituent parts A and B. It's significant to consider that while synthesis reactions often release energy, decomposition reactions usually absorb energy from their surroundings to initiate and sustain the reaction.
Decomposition Reaction: A type of chemical reaction where a single compound undergoes a reaction that results in two or more simpler products.
A classic example of a decomposition reaction involves the breakdown of calcium carbonate when heated, producing calcium oxide and carbon dioxide gas:
CaCO3(s) ightarrow CaO(s) + CO2(g)
Decomposition reactions are integral in various biological processes, including digestion and decomposition, aiding in the transfer and cycling of matter.
The decomposition of hydrogen peroxide into water and oxygen is another important example. This reaction proceeds slowly at room temperature but can be accelerated by the addition of a catalyst such as manganese dioxide. The reaction is essential in biological systems where enzymes like catalase perform this decomposition to protect cells from the harmful effects of hydrogen peroxide.
Single and Double Displacement: A Comparison
Displacement reactions refer to chemical reactions where ions or atoms in a compound are replaced by ions or atoms of another element. There are two main types: single displacement and double displacement reactions. In a single displacement reaction, an element reacts with a compound, displacing another element to form a new compound and releasing the displaced element. In contrast, a double displacement reaction involves two compounds exchanging ions or atoms to form two new compounds.
Single Displacement Reaction: A reaction in which an element reacts with a compound, displacing an element from the compound to form a new element and compound.
Double Displacement Reaction: A reaction where two ionic compounds exchange ions to form two new compounds.
For a single displacement reaction:
Zn(s) + CuSO4(aq) ightarrow ZnSO4(aq) + Cu(s)
And for a double displacement reaction:
AgNO3(aq) + NaCl(aq) ightarrow AgCl(s) + NaNO3(aq)
A helpful way to remember these reactions is that in a single displacement reaction, 'A' pushes 'B' away, whereas in a double displacement reaction, 'A' and 'C' swap places.
Double displacement reactions are often characterised by the formation of a precipitate, a gas, or a weak electrolyte when two solutions are mixed. This is notably visible in the reaction between sodium carbonate and calcium chloride, where calcium carbonate, a white precipitate, is formed along with sodium chloride in solution.
It's important to understand that not all potential displacement reactions will occur. Whether a single displacement reaction takes place can be predicted using the reactivity series, which orders metals by their reactivity. A metal will displace another in a compound only if it is above the displaced metal in the series.
Chemical equations are symbolic representations of chemical reactions that describe the transformation of reactants into products, encapsulating the law of conservation of mass. They consist of chemical formulas and symbols that denote the quantities and substances involved in the reaction, with the balancing of these equations being a critical procedural step. The balanced equation ensures that an equal number of atoms for each element is present on both sides, highlighting the cause-and-effect relationship intrinsic to chemical processes.
Chemical Equations and Reactions: Cause and Effect
At the heart of chemistry lies the ability to understand and represent how substances transform and interact with one another. Chemical equations and reactions epitomise this cause-and-effect relationship in chemical processes, illustrating the before-and-after states of a reaction, from the initial reactants to the final products.
Linking Reactants to Products through Equations
When chemical reactions occur, the reactants - the starting materials - undergo physical and chemical changes to become new substances, known as products. Chemical equations are the linguistic bridge that connects these two states, providing a concise and accurate method to portray this transformation. They serve as the language through which chemists communicate and predict the outcomes of reactions, allowing for both quantification and visualisation of the process. Understanding how to link reactants to products through equations begins with the identification of the substances involved. A typical chemical equation lists the reactants on the left, the products on the right, and employs an arrow ( ightarrow) to indicate the direction of the transformation. For a complete and informative depiction, additional details, such as physical states, heat changes, and catalysts, may also be included. Adeptly linking reactants to products requires an understanding of certain rules and conventions, such as stoichiometry, which involves the ratio of reactants to products based on moles. It also encompasses the study of reaction mechanisms, detailing the concise step-by-step process by which reactants are transformed into products on a molecular level. Through mastering these elements, one can not only construct and balance a chemical equation but also decipher the intricate narrative it tells.
Stoichiometry: A section of chemistry that deals with the relative quantities of reactants and products in chemical reactions. It is founded on the law of conservation of mass and helps in calculating the amounts of substances required or produced during a reaction.
An example of a simple chemical reaction and equation is the formation of water from hydrogen and oxygen gas:
2H2(g) + O2(g) ightarrow 2H2O(l)
The equation shows the reactants (hydrogen and oxygen) and the product (water) while indicating the mole ratio and physical states of each substance involved.
Remember that the conservation of mass dictates that matter cannot be created or destroyed in a chemical reaction, which is reflected in the equal number of atoms of each element appearing on both sides of a balanced chemical equation.
Linking reactants to products extends beyond the mere balancing of an equation. It delves into the underlying principles that govern chemical reactions, such as thermodynamics, which predicts whether a reaction is energetically favourable, and kinetics, which describes the speed of a reaction. Understanding these principles contributes to grasping how certain conditions can influence the direction and extent of a chemical transformation and how this is accurately reflected in the corresponding chemical equation.
Predicting the Products of Chemical Reactions
Predicting the products of chemical reactions is a fundamental skill in chemistry that involves logical deductions based on an understanding of elements and compounds, as well as the recognition of reaction patterns. With this knowledge, scientists can forecast the outcome of reactions before they actually occur in the laboratory or industrial processes. This foresight is invaluable in designing experiments, creating new materials, and ensuring safety in chemical handling.Generally, predictions start with the identification of reactant types. Are they elements, simple compounds, ionic compounds, or organic molecules? Next, one should consider the reaction environment, which includes the temperature, pressure, concentration of reactants, and the presence of catalysts or inhibitors. Based on this information, along with a firm grasp of recognized reaction types (such as synthesis, decomposition, single replacement, or combustion), one can make educated guesses about the products. However, there are also sophisticated models and computational methods that can predict products with greater precision. Quantum chemistry calculations, for example, utilise fundamental principles of physics to determine the most stable configurations of atoms following a reaction. Moreover, systematic experimentation and analysis, guided by hypotheses about possible reaction pathways, can lead to the anticipation of products even in novel or complex reactions.
Reaction Pathway: A detailed description of the step-by-step sequence of events at the molecular level that leads from reactants to products in a chemical reaction.
For instance, when predicting the products of a reaction between an acid and a base, one can anticipate the formation of water and a salt as the result of a neutralisation reaction:
HCl(aq) + NaOH(aq) ightarrow H2O(l) + NaCl(aq)
This is based on an understanding of the characteristic reaction patterns of acids and bases.
The activity series of metals, which ranks metals based on their reactivity, can provide guidance when predicting the products of single replacement reactions; a more reactive metal will replace a less reactive metal in a compound.
In research and industry, predicting reaction products has applications that reach far beyond academic inquiry. For instance, in pharmaceutical drug design, knowing how certain compounds interact with biological enzymes and receptors helps in the development of new medicines. In environmental science, predicting reaction products enables the forecasting of pollution pathways and the design of effective remediation strategies. As such, the ability to anticipate the results of chemical reactions is essential to technological and scientific advances across disciplines.
Chemical equations encapsulate the essence of chemistry, providing a symbolic representation of how substances interact to form new compounds. To comprehend these equations fully, one must delve into the laws of balancing chemical equations, ensuring equitable atoms on both sides for each element. This balance is a direct application of the law of conservation of mass. Chemical equations are constructed using formulas to denote the substances and include symbols that illustrate their physical state, reaction conditions, and the direction of change from reactants to products.
Deciphering the Chemical Equations Formula
Deciphering the formula of a chemical equation is akin to translating a complex language into a universal scientific narrative, providing insights into the nature and outcome of chemical reactions. In chemistry, the ability to read and understand these formulas is paramount as it explains the stoichiometry and dynamics of reactants turning into products. The intricacies involved in decoding a chemical equation require a comprehensive understanding of chemical symbols, the balancing act to maintain mass conservation, and the ability to interpret the additional information couched within the equation.
Constructing a Chemical Equation Step-by-Step
Creating a chemical equation requires meticulous attention to detail and a systematic approach. It is an art as much as it is a science. To achieve an accurate and balanced equation, you must consider both the qualitative and quantitative aspects of the reactants and products involved. Here’s how you begin:
- Step 1: Identify reactants and products – Begin by writing down the reactant(s) on the left and the product(s) on the right-hand side of the equation.
- Step 2: Write correct formulae – Ensure that you have the correct chemical formulae for each of the reactants and products based on their chemical bonds and structure.
- Step 3: Balance atoms – Adjust the coefficients in front of the formulae to balance the number of atoms of each element on both sides of the equation, maintaining the law of conservation of mass.
- Step 4: Include state symbols – Put the appropriate state symbols (solid, liquid, gas, aqueous) after each formula to provide context on the physical state of each substance.
- Step 5: Add reaction conditions – Specify conditions such as temperature, pressure or the presence of catalysts below or above the reaction arrow if they are not standard conditions.
- Step 6: Review and refine – Finally, review the balanced equation and make sure that all coefficients are in their simplest whole number form, if possible. Ensure that the equation reflects the actual reaction process accurately.
Throughout this process, it's important to cross-check the count of each atom on both sides of the equation. If any discrepancies are found, go back and reassess the coefficients until balance is achieved. Remember that it's only the coefficients that can be altered to balance an equation; the subscripts in chemical formulas must remain unchanged because they define the compound itself.
Coefficients: Numbers placed before the chemical formulae in an equation to indicate the ratio of molecules or moles of each substance involved in the reaction.
Constructing an equation for the combustion of propane (C3H8) in oxygen might look like this:
C3H8 + O2 ightarrow CO2 + H2O
This initial unbalanced equation then needs to be balanced, leading to:
C3H8 + 5O2 ightarrow 3CO2 + 4H2O
Here, the coefficients 1 (implied for C3H8), 5, 3, and 4 ensure that each element is balanced across reactants and products.
A practical tip while balancing chemical equations is to save elements that appear in multiple reactants or products, such as oxygen and hydrogen, for last, as their ratios are more flexible and they can balance out remaining discrepancies.
When looking deeper into reaction conditions, it's important to note that some reactions will not proceed at a measurable rate without the presence of a catalyst, while others might only occur under specific temperatures or pressures. These conditions don’t appear in the chemical formulas but are essential for actually carrying out the reaction and must be provided alongside the equation.
Real-world Examples of Chemical Equations Formulas in Use
Real-world applications of chemical equations are abundant and diverse, spanning many sectors from industrial manufacturing to environmental sciences and biological processes. Understanding and modelling these reactions is essential for industries to produce chemicals and materials efficiently, and for scientists to predict environmental impacts and biological changes. For instance, the chemical equation for synthesising ammonia (NH3) through the Haber process is a fundamental reaction in the production of fertilisers:
N2(g) + 3H2(g) ightleftharpoons 2NH3(g)
This reversible reaction occurs under high pressure and with a catalyst, reflecting its balanced equation in the real conditions it requires. In pharmacology, equations guide the synthesis of drugs. The creation of aspirin (C9H8O4) from salicylic acid (C7H6O3) involves:
C7H6O3 + C4H6O3 ightarrow C9H8O4 + C2H4O2
While environmental processes, such as the breakdown of ozone (O3), are represented by the equation:
2O3(g) ightarrow 3O2(g)
This reaction is critical to understanding ozone depletion in the Earth's atmosphere. Photosynthesis in plants, the process that converts sunlight into chemical energy, is also illustrated by a chemical equation, where carbon dioxide and water yield glucose and oxygen in the presence of sunlight:
6CO2(g) + 6H2O(l) ightarrow C6H12O6(s) + 6O2(g)
Each of these equations not only provides a recipe for the reactants and products involved but also a wealth of information regarding the conditions needed and the stoichiometry of the substances as the reaction moves forward.
Stoichiometry: It is the aspect of chemistry that pertains to the quantitative relationships between reactants and products in a chemical reaction.
Consider the chlorination of methane (CH4) which produces methyl chloride (CH3Cl) and hydrogen chloride (HCl), a reaction significant in organic synthesis:
CH4(g) + Cl2(g) ightarrow CH3Cl(g) + HCl(g)
When utilising chemical equations in real-world scenarios, consider that many reactions proceed via multiple steps and may involve intermediate products not detailed in the final balanced equation.
The intricate process of wine fermentation illustrates a real-world example where multiple reactions occur simultaneously. The primary equation, showing the conversion of glucose into ethanol and carbon dioxide, is:
C6H12O6(s) ightarrow 2C2H5OH(l) + 2CO2(g)
This process, catalysed by enzymes in yeast cells, has tremendous economic significance and showcases the complex interplay of both biological and chemical factors that can be captured by chemical equations.
Chemical Equations - Key takeaways
- A chemical equation is a symbolic representation of a chemical reaction, depicting the transformation of reactants into products while showcasing quantitative relationships.
- Balancing chemical equations is essential to ensure the conservation of mass as per the law of conservation of matter, making the number of atoms of each element equal on both sides of the equation.
- Common types of chemical equations include synthesis, decomposition, single replacement, and double replacement reactions, each with distinct reactant and product patterns.
- The formulae and symbols within chemical equations communicate specific information, such as the state of reactants and products, the amount of each substance, energy changes, and reaction conditions.
- Mastering the chemical equations formula requires understanding symbols, balancing equations for mass conservation, and interpreting additional details like physical states and reaction conditions.
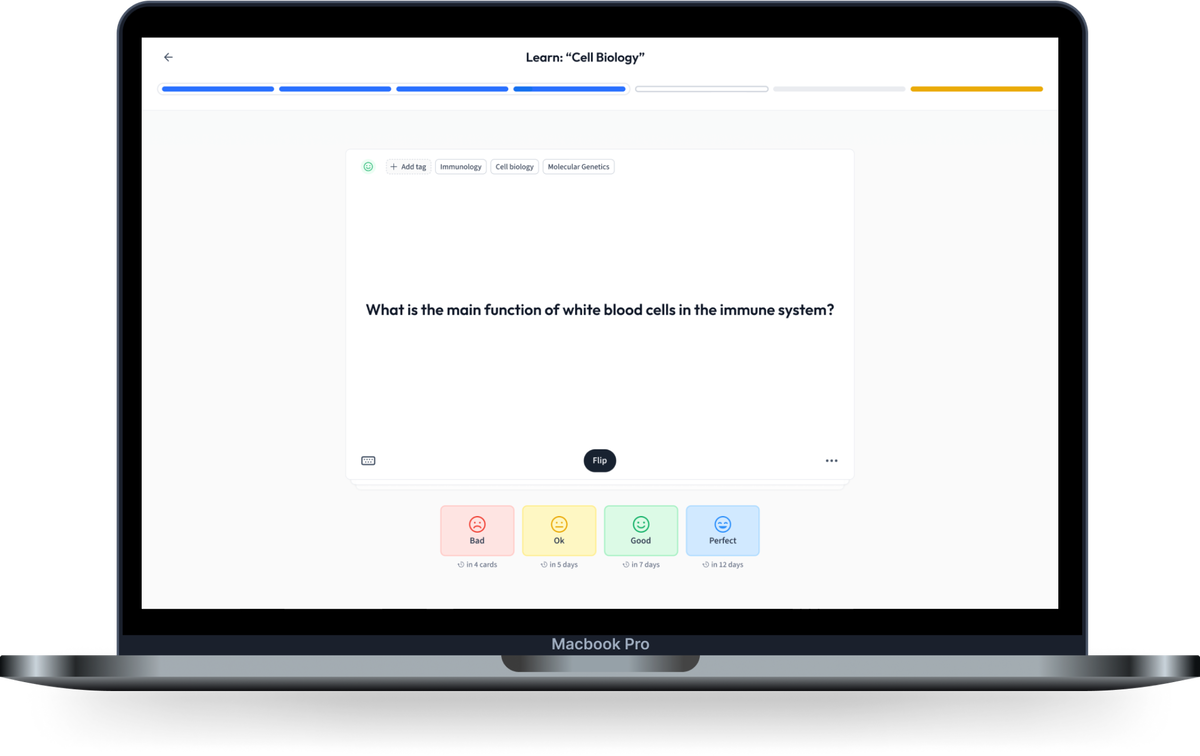
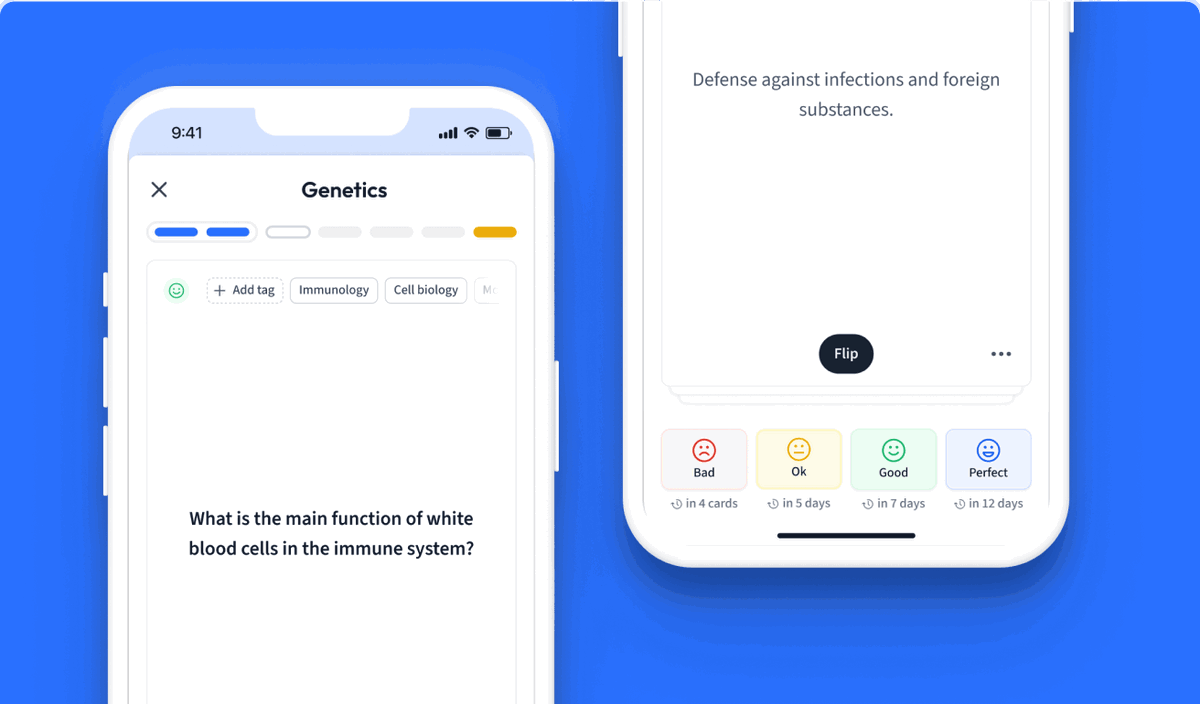
Learn with 15 Chemical Equations flashcards in the free StudySmarter app
We have 14,000 flashcards about Dynamic Landscapes.
Already have an account? Log in
Frequently Asked Questions about Chemical Equations
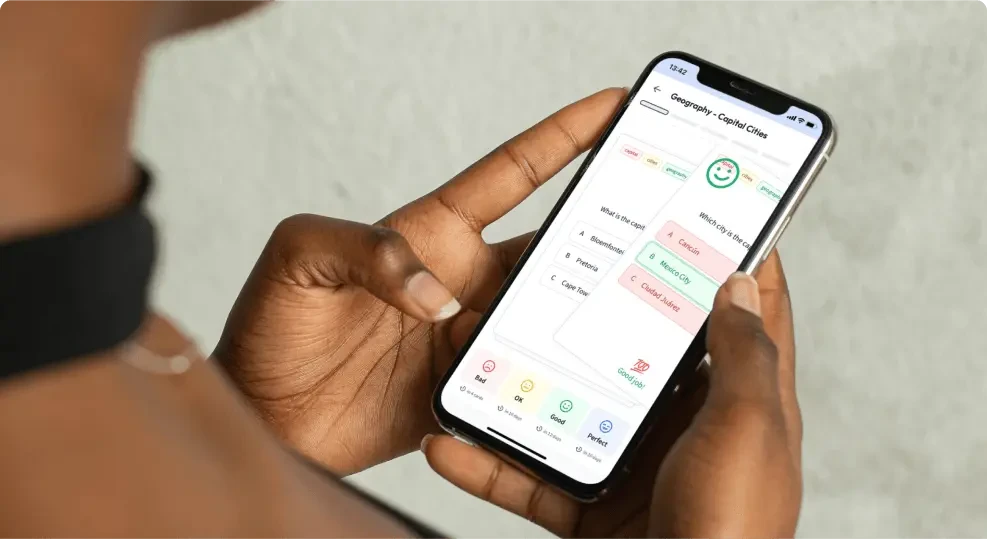
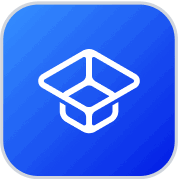
About StudySmarter
StudySmarter is a globally recognized educational technology company, offering a holistic learning platform designed for students of all ages and educational levels. Our platform provides learning support for a wide range of subjects, including STEM, Social Sciences, and Languages and also helps students to successfully master various tests and exams worldwide, such as GCSE, A Level, SAT, ACT, Abitur, and more. We offer an extensive library of learning materials, including interactive flashcards, comprehensive textbook solutions, and detailed explanations. The cutting-edge technology and tools we provide help students create their own learning materials. StudySmarter’s content is not only expert-verified but also regularly updated to ensure accuracy and relevance.
Learn more