- This article is about the electrophilic substitution of benzene.
- We'll start with an overview of benzene electrophilic substitution reactions before thinking about why benzene takes part in substitution reactions.
- We'll then learn the general equation and mechanism for the electrophilic substitution of benzene.
- After that, we'll practice applying the general mechanism to examples of benzene electrophilic substitution.
- To finish the article, we'll explore directing effects involved in the electrophilic substitution of benzene.
Benzene electrophilic substitution reactions
Electrophilic substitution reactions are reactions in which one atom, group of atoms or functional group is replaced by another on a molecule. The reaction is triggered by an electrophile, which is an electron-pair acceptor with an empty electron orbital and a positive or partially-positive charge.
You might already be familiar with the organic molecule benzene (C6H6). It is an example of an aromatic compound: a molecule with a ring of delocalised pi electrons. In particular, benzene has six delocalised electrons, but other aromatic compounds may have more or fewer. Aromatic molecules are also known as arenes.
Check out Aromatic Chemistry for an overview of benzene and other aromatic molecules.
Benzene frequently takes part in electrophilic substitution reactions, which we defined above. In these reactions, benzene is attacked by an electrophile. Consequently, one of benzene's hydrogen atoms is replaced by a different functional group such as a halogen atom, hydroxyl group, or nitrate group.
Typical electrophilic substitution reactions of benzene include:
- Nitration.
- Halogenation (such as chlorination or bromination).
- Friedel-Crafts acylation.
- Friedel-Crafts alkylation.
Each of these reactions has three steps:
The electrophile is generated.
The electrophile reacts with benzene using the general benzene electrophilic substitution mechanism.
The catalyst is regenerated.
But before we delve any further into the world of electrophilic substitution reactions of benzene, let's think about why benzene takes part in this type of reaction.
Why does benzene undergo substitution reactions?
As we mentioned earlier, benzene is an aromatic molecule. All aromatic molecules are characterised by their ring of delocalised pi electrons. The ring of delocalisation is an area of high electron density - after all, it contains six free-moving delocalised electrons! This ring is particularly attractive to electrophiles, which are electron-pair acceptors, and so benzene commonly finds itself under electrophilic attack.
However, the aromatic ring is relatively strong and stable because it spreads the charge of the six delocalised electrons over a greater area. It takes a lot of energy to disrupt the delocalisation. Therefore, benzene electrophilic attack doesn't usually result in reactions that involve breaking the ring by forming additional bonds with the delocalised electrons - which is exactly the case in addition reactions. Instead, attack from electrophiles often results in substitution. Substitution keeps the delocalised electron ring intact and so it is a lot more favourably energetic for benzene than addition. This is why benzene prefers to take part in electrophilic substitution reactions.
Head over to Reactions of Alkenes for a different type of reaction involving electrophiles: electrophilic addition reactions.
Electrophilic substitution of benzene equation
We now know what an electrophilic substitution reaction is. Let's learn the general equation for an electrophilic substitution reaction of benzene.
$$C_{6}H_{6} + El^{+}\rightarrow C_{6}H_{5}El + H^{+}$$
In electrophilic substitution reactions, benzene (C6H6) reacts with an electrophile (El+) to produce a benzene derivative (C6H5El) and a hydrogen ion (H+). The benzene derivative is much like benzene except one of its hydrogen atoms is replaced by the electrophile.
Electrophilic substitution of benzene mechanism
It's now time to look at the general mechanism of an electrophilic substitution reaction of benzene.
Here, the electrophile is represented by El+.
Fig. 1: The general mechanism of benzene electrophilic substitution.StudySmarter Originals
The electrophile is attracted to benzene’s aromatic ring of delocalisation. The electrophile forms a bond with one of benzene’s carbon atoms, using two electrons from the aromatic ring. This partially destroys the ring of delocalisation and leaves benzene with a positive charge.
Benzene would be a lot more stable if it repaired its disrupted electron ring by replacing the two lost delocalised electrons. To do this, one of its C-H bonds breaks heterolytically. Both electrons go to the ring of delocalisation, restoring it back to six electrons, and the hydrogen atom is lost as a hydrogen ion (H+).
The final organic product is a benzene derivative with the electrophile in place of one of its hydrogen atoms. We also end up with a hydrogen ion.
Here are a couple of things you should pay attention to:
- Notice that in the second step, benzene's disrupted electron ring has four delocalised electrons spread over five carbon atoms. This leaves it with a positive charge.
- To show the disrupted benzene ring, we draw a broken circle. Remember to include the positive charge in the center of the circle.
- It isn't just any C-H bond that breaks - make sure that the hydrogen ion lost comes from the carbon atom with the new C-El bond!
We'll now practice applying this mechanism to the four benzene electrophilic substitution reactions we mentioned earlier: nitration, halogenation, Friedel-Crafts acylation, and Friedel-Crafts alkylation.
Electrophilic substitution of benzene examples
We covered examples of electrophilic substitution reactions of benzene in the article Reactions of Benzene. In that article, you learned about the reactants and conditions for various different benzene electrophilic substitution reactions. We'll visit these examples again, but this time we'll also focus on the reaction mechanism. First up: nitration.
Nitration of benzene
We nitrate benzene by heating it under reflux at 50 °C with a mixture of concentrated sulphuric and nitric acids (H2SO4 and HNO3). The sulphuric acid acts as a catalyst. It is used to generate our electrophile, which is the nitronium ion (NO2+), but is reformed at the end of the reaction. Here's the equation for the formation of NO2+:
$$H_{2}SO_{2} + HNO_{3}\rightarrow NO_{2}^{+} + HSO_{4}^{+} + H_{2}O $$
The nitronium ion electrophile NO2+ then reacts with benzene in an electrophilic substitution reaction using the general mechanism that we learned above. The reaction swaps one of benzene's hydrogen atoms for the nitrate group (-NO2) and releases a hydrogen ion (H+). The hydrogen ion then reacts with the HSO4- produced when we generated our electrophile to reform the sulphuric acid catalyst.
$$H^{+} + HSO_{4}^{-} \rightarrow H_{2}SO_{4}$$
Overall, we produce nitrobenzene (C6H5NO2) and water. The reaction has the following equation:
$$C_{6}H_{6}+ HNO_{3} \rightarrow C_{6}H_{5}NO_{2} + H_{2}O$$
Now it's your turn - practice applying the general electrophilic substitution mechanism to the nitration of benzene. Our worked example below will help you if you get stuck.
Draw the mechanism for the nitration of benzene using a mixture of concentrated sulphuric and nitric acids. State the conditions needed for the reaction.
Fig. 2: The mechanism for the nitration of benzene.StudySmarter Originals
Our electrophile is the nitronium ion (NO2+), which is attracted to benzene's aromatic ring and forms a C-NO2 bond using two of benzene's delocalised electrons. This disrupts the aromatic ring and causes a C-H bond to break heterolytically. Both electrons return to the aromatic ring, restoring its stability, and the hydrogen atom is released as a hydrogen ion (H+).
The reaction uses a mixture of concentrated sulphuric and nitric acids and takes place at 50 °C under reflux.
Halogenation of benzene
Benzene is halogenated using a halogen (X2) and its corresponding aluminium halide (AlX3). For example, chlorination uses chlorine gas (Cl2) and aluminium chloride (AlCl3), whilst bromination uses bromine water (Br2) and aluminium bromide (AlBr3). The aluminium halides act as catalysts. Alternatively, you can catalyse the reactions using iron(III) halides (FeCl3 or FeBr3 respectively). Benzene halogenation is another type of electrophilic substitution reaction and uses the same general mechanism as nitration. All the benzene halogenation reactions take place at room temperature.
Our electrophile is a positive halogen cation. It is generated by reacting the aluminium halide with the halogen. This also forms a negative aluminium tetrahalide ion (AlX4-). Here's the equation:
$$X_{2} + AlX_{3}\rightarrow X^{+} + AlX_{4}^{-}$$
The halogen cation then reacts with benzene using the general mechanism we learned earlier. This forms a halogenoarene (C6H5X) and a hydrogen ion (H+). The hydrogen ion reacts with the negative aluminium tetrahalide ion we produced when generating the electrophile to produce a hydrogen halide (HX) and reform the catalyst (AlX3):
$$H^{+} + AlX_{4}^{-} \rightarrow AlX_{3} + HX$$
Overall, we form a halogenoarene (C6H5X) and a hydrogen halide (HX) using the following equation:
$$C_{6}H_{6} + X_{2} \rightarrow C_{6}H_{5}X + HX$$
Draw the mechanism for the bromination of benzene using a mixture of bromine water and aluminium bromide. State the conditions needed for the reaction and write an overall equation.
Fig. 3: The mechanism for the bromination of benzene.StudySmarter Originals
Our electrophile is the positive bromine cation (Br+), which is attracted to benzene's aromatic ring and forms a C-Br bond with two of benzene's delocalised electrons. This disrupts the aromatic ring and causes a C-H bond to break heterolytically. Both electrons return to the aromatic ring, restoring its stability, and the hydrogen atom is released as a hydrogen ion (H+).
The reaction takes place at room temperature, using bromine water and an aluminium bromide (AlBr3) catalyst.
$$C_{6}H_{6} + Br_{2}\rightarrow C_{6}H_{5}X + HBr$$
What happens if we try to halogenate an alkylarene? Under the conditions explored above - at room temperature and with an aluminium halide catalyst - the reaction substitutes a halogen atom into the benzene ring. However, under different conditions, we can instead substitute the halogen atom into the alkyl chain. This requires UV light and is an example of free radical substitution, which we cover in Chlorination. We'll practice deciding between both types of substitution later on in the article.
Friedel-Crafts acylation of benzene
One method of acylating benzene involves reacting benzene with a mixture of an acyl chloride (RCOCl) and aluminium chloride (AlCl3) in a further example of a benzene electrophilic substitution reaction. The reaction takes place in anhydrous conditions under reflux. Here, aluminium chloride acts as a catalyst and is used to generate the electrophile, which in this reaction is the positive RCO+ cation. Notice that the other product of the electrophile generation is once again a negative aluminium tetrachloride ion (AlCl4-).
$$RCOCl + AlCl_{3} \rightarrow RCO^{+} + AlCl_{4} ^{-}$$
The RCO+ electrophile reacts with benzene using the same mechanism as all of our other examples. Again, it releases a hydrogen ion. The hydrogen ion reacts with the negative aluminium tetrachloride ion (AlCl4-) to produce hydrogen chloride (HCl) and regenerate the catalyst (AlCl3):
$$H^{+} + AlCl_{4}^{-} \rightarrow AlCl_{3} + HCl$$
Overall, the reaction produces a ketone with a benzene ring attached (C6H5COR - this is also known as an aromatic ketone) and hydrogen chloride (HCl). We use the prefix phenyl- to name the organic ketone.
$$C_{6}H_{6} + RCOCl \rightarrow C_{6}H_{5}COR + HCl $$
Draw the mechanism for the reaction between propanoyl chloride and benzene. State the reaction conditions and give the equation for the overall reaction.
Fig. 4: The mechanism for the acylation of benzene using propanoyl chloride.StudySmarter Originals
Our electrophile is the positive CH3CH2CO+ ion. It is attracted to benzene's aromatic ring and forms a bond with a carbon atom using two of benzene's delocalised electrons. This disrupts the aromatic ring and causes a C-H bond to break heterolytically. Both electrons return to the aromatic ring, restoring its stability, and the hydrogen atom is released as a hydrogen ion (H+).
The reaction uses propanoyl chloride (CH3CH2COCl) and an aluminium chloride (AlCl3) catalyst, and takes place in anhydrous conditions under reflux.
$$C_{6}H_{6} + CH_{3}CH_{2}COCl \rightarrow C_{6}H_{5}COCH_{2}CH_{3} + HCl$$
Can you name the ketone formed? It is 1-phenylpropan-1-one. Check out IUPAC Nomenclature for more about naming organic compounds.
Friedel-Crafts alkylation of benzene
The last example of electrophilic substitution of benzene that we'll look at today is Friedel-Crafts alkylation. In this reaction, we swap one of benzene's hydrogen atoms for an alkyl group using a mixture of a chloroalkane (RCl) and aluminium chloride (AlCl3). Aluminium chloride is once again a catalyst and helps generate the electrophile, which is a positive R+ carbocation:
RCl + AlCl3 → R+ + AlCl4-
The R+ carbocation then reacts with benzene using the same general electrophilic substitution mechanism as before to produce an alkylarene (C6H5R) and a hydrogen ion (H+). The hydrogen ion reacts with the aluminum tetrachloride (AlCl4-) produced when generating the electrophile to form hydrogen chloride (HCl) and revive our catalyst (AlCl3). Here's the equation for the regeneration of the catalyst:
$$H^{+} + AlCl_{4}^{-} \rightarrow AlCl_{3} + HCl $$
Overall, we produce an alkylarene (C6H5R) and hydrogen chloride (HCl).
$$C_{6}H_{6} + RCl \rightarrow C_{6}H_{5}R + HCl$$
Have a go at drawing the mechanism for this next example.
Draw the mechanism for the reaction between chloroethane and benzene. Give the conditions and equation for the overall reaction.
Fig. 5:The mechanism for the alkylation of benzene using chloroethane.StudySmarter Originals
Our electrophile is the positive CH3CH2+ ion, which is attracted to benzene's aromatic ring and forms a bond with a carbon atom using two of benzene's delocalised electrons. This disrupts the aromatic ring and causes a C-H bond to break heterolytically. Both electrons return to the aromatic ring, restoring its stability, and the hydrogen atom is released as a hydrogen ion (H+).
The reaction uses chloroethane (CH3CH2Cl) and an aluminium chloride (AlCl3) catalyst.
$$C_{6}H_{6} + CH_{3}CH_{2}Cl \rightarrow C_{6}H_{5}CH_{2}CH_{3} + HCl$$
This organic product is known as ethylbenzene.
Directing effects of the electrophilic substitution of benzene
In all of the examples we've investigated so far, we've only made one substitution into the aromatic benzene ring. However, if you provide an excess of the electrophile, you can actually repeat the reaction over and over again, substituting many of benzene's hydrogen atoms for other functional groups.
The first substitution of benzene is always random. After all, benzene's carbon and hydrogen atoms are identical - the chances of the electrophile attacking any one of its carbon atoms are equal. We call the carbon atom with the newly-substituted functional group carbon 1.
But subsequent substitutions aren't random. This is because some functional groups attached to the benzene ring have specific directing effects. They encourage the next electrophile to attack a certain carbon atom. Here are their effects.
- Electron-donating groups encourage the next electrophile to attack carbon atoms 2, 4, and 6in the benzene ring. These groups include:
- Alkyl groups (-R).
- The hydroxyl group (-OH).
- The amine group (-NH2).
- The ketone carbonyl group (-COR).
- Electron-withdrawing groups encourage the next electrophile to attack carbon atoms 3 and 5in the benzene ring. These groups include:
- The nitrate group (-NO2).
- The carboxyl group (-COOH).
Now have a go at applying your knowledge about the directing effect to some practice questions.
Predict the final product formed when benzene reacts under reflux with an excess of concentrated sulphuric and nitric acids.
This reaction is an electrophilic substitution nitration reaction. It swaps some of benzene's hydrogen atoms for nitrate groups (-NO2). We call the carbon atom with the first substituted nitrate group carbon 1; this new nitrate group then affects the positions of subsequent substitutions. The nitrate group is electron-withdrawing and so directs the next nitronium ion electrophiles to attack carbon atoms 3 and 5 in the benzene ring. If we leave the reaction for long enough, eventually both substitutions take place. We therefore end up with 1,3,5-trinitrobenzene.
Fig. 6: The final result of successive nitrations of benzene due to the directing effects of the nitrate group.StudySmarter Originals
Now try this second example. It draws on the information we learned earlier about substitution involving halogen atoms.
Draw the likely product(s) formed when methylbenzene takes part in one further substitution reaction with:
- Chlorine gas and aluminium chloride, at room temperature.
- Chlorine gas, under UV light.
Notice the conditions given in the two parts of this question. In part a), the conditions favour electrophilic substitution into the benzene ring. We know that methylbenzene already contains an alkyl group joined to the benzene ring. Alkyl groups are electron-donating and so direct the electrophile to attack carbon atoms 2, 4, or 6, giving us the following possible organic products:
Fig. 7: The possible products of chlorination of methylbenzene due to the directing effects of the methyl group.StudySmarter Originals
Note that the molecule on the left and the molecule on the right are actually one and the same - carbon 6 is simply carbon 2 if you count the chain in the other direction!
On the other hand, in part b), the conditions favour free radical substitution of the methyl group already present in methylbenzene. We therefore end up with the following organic product, (chloromethyl)benzene:
Fig. 8: The product of free radical substitution of methylbenzene.StudySmarter Originals
Be careful when naming the potential products of part a) in the example above. These molecules contain both chlorine atom and methyl group substituents and so need the prefixes chloro- and methyl-. However, chloro- comes before methyl- in the alphabet and so one of the chlorine substituents must always be found on carbon 1 - not the methyl group! According to IUPAC nomenclature:
- The molecule on the left is systematically known as 1-chloro-2-methylbenzene.
- The molecule in the middle is systematically known as 1-chloro-4-methylbenzene.
- The molecule on the right, which we know is the same as the molecule on the left, is obviously also systematically known as 1-chloro-2-methylbenzene.
You might also notice the brackets in the name of the product in part b). We include these to make it extra clear that the chlorine atom is part of the methyl group, instead of being directly attached to the benzene ring.
Electrophilic Substitution Of Benzene - Key takeaways
- Benzene is an aromatic compound with the formula C6H6.
- Benzene frequently takes part in electrophilic substitution reactions. In these reactions, one atom, group of atoms or functional group is replaced by another on a molecule as a result of an electrophilic attack.
- Benzene resists addition reactions because of its stable ring of delocalised electrons.
- Common electrophilic substitution reactions of benzeneinclude:
- Nitration, producing nitrobenzene.
- Halogenation, producing a halogenoarene.
- Acylation, producing an aromatic ketone.
- Alkylation, producing an alkylarene.
- All benzene electrophilic substitution reactions follow the same general mechanism and have the general equation C6H6 + El+ → C6H5El + H+.
- When it comes to halogen substitution reacts of alkylarenes, the reaction conditions determine whether the substitution takes place in the benzene ring or in the alkyl group.
- Some functional groups have directing effects and dictate the position of electrophilic attack in further substitution reactions.
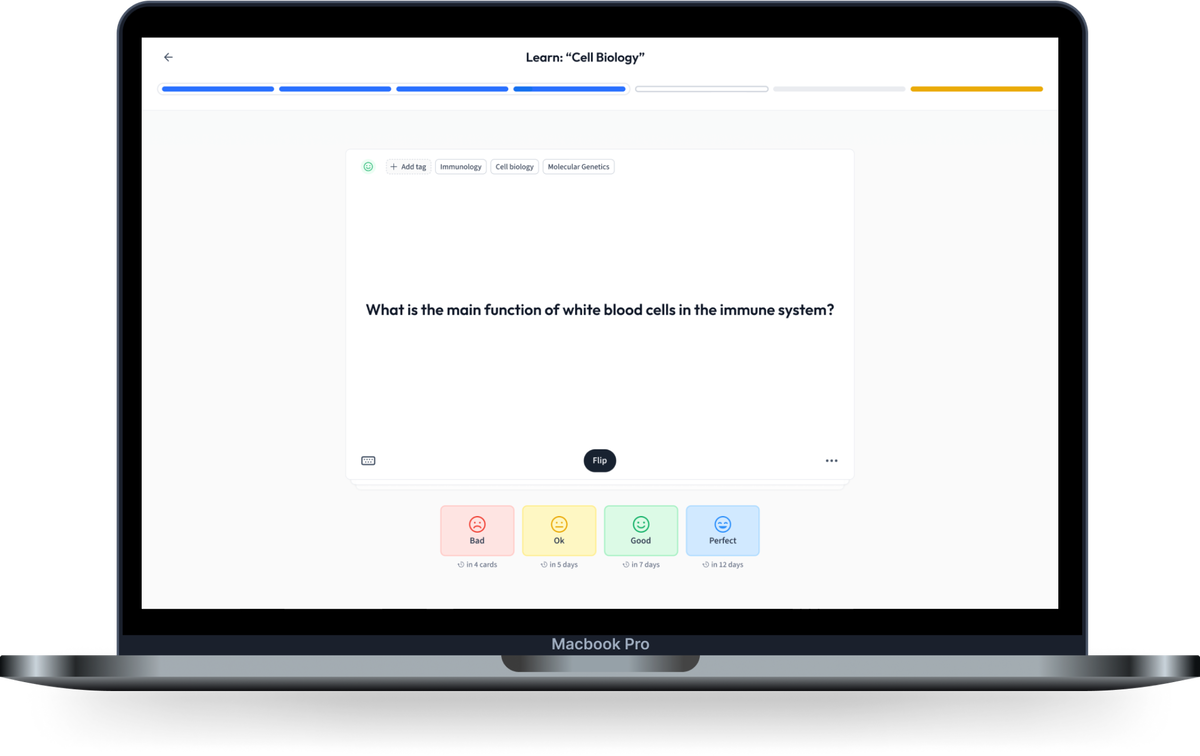
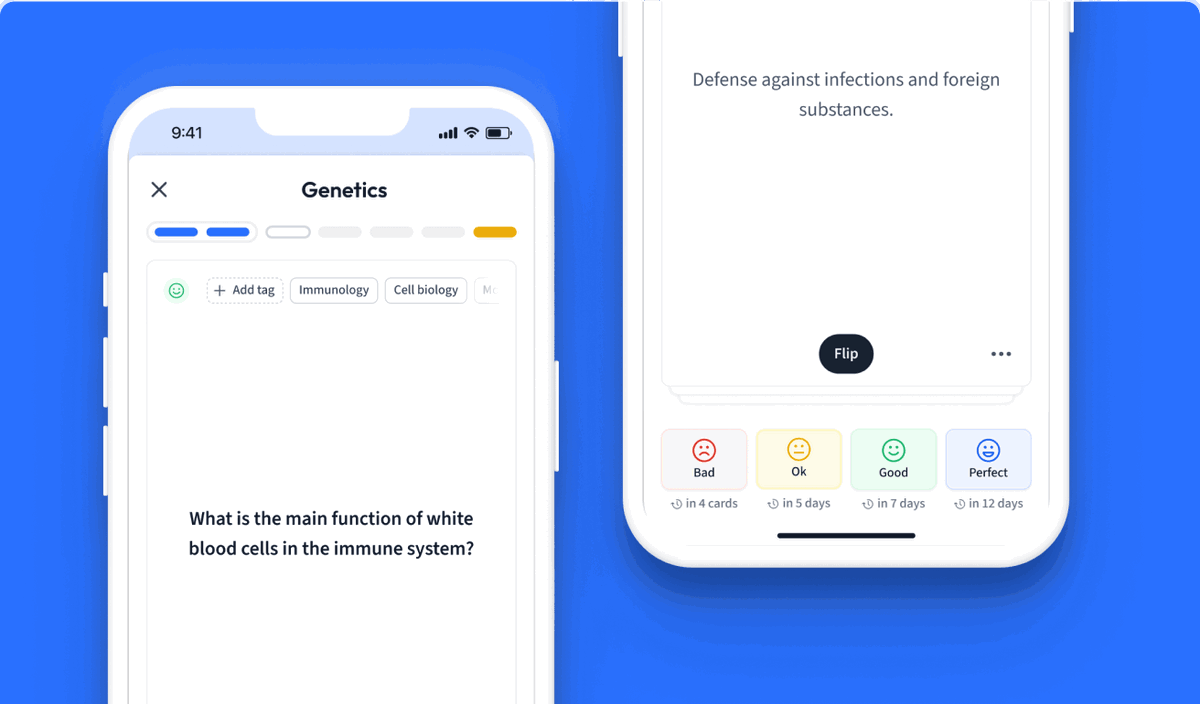
Learn with 17 Electrophilic Substitution of Benzene flashcards in the free StudySmarter app
We have 14,000 flashcards about Dynamic Landscapes.
Already have an account? Log in
Frequently Asked Questions about Electrophilic Substitution of Benzene
What are electrophilic substitution reactions of benzene?
Electrophilic substitution reactions are reactions in which one atom, group of atoms or functional group is replaced by another on a molecule. The reaction is triggered by an electrophile, which is an electron-pair acceptor with an empty electron orbital and a positive or partially-positive charge. Benzene reacts in a vast number of different electrophilic substitution reactions, including nitration, halogenation, and Friedel-Crafts reactions. In all of these reactions, an electrophile attacks benzene's aromatic ring of delocalised electrons and replaces one of the molecule's hydrogen atoms.
What are the conditions for electrophilic substitution?
Different electrophilic substitution reactions of benzene have different conditions. For example, nitration requires mixing concentrated sulphuric and nitric acids together with benzene and heating at 50 °C under reflux. On the other hand, chlorination requires combining chlorine gas and aluminium chloride with bromine and leaving the reaction vessel at room temperature.
Why does benzene undergo electrophilic substitution reactions instead of addition reactions?
Benzene is very attractive to electrophiles because of its ring of delocalised electrons, which is an area of high electron density. However, benzene doesn't tend to take part in addition reactions because this would involve forming bonds with its delocalised electrons and disrupting the ring's stability. Addition reactions simply require too much energy. Instead, benzene reacts in substitution reactions. These reactions keep the delocalised electron intact and are much more energetically favourable.
Which catalyst is used in electrophilic substitution?
The catalyst used for electrophilic substitution depends on the reaction. For example, nitration uses concentrated sulphuric acid as a catalyst, whilst halogenation uses an aluminium halide. Friedel-Crafts acylation and alkylation both use aluminium chloride to catalyse their reactions.
Does benzene need a catalyst?
Benzene electrophilic substitution reactions generally require a catalyst in order to form the electrophile. The catalyst helps generate a positive cation that is strong enough to attack the stable benzene ring successfully.
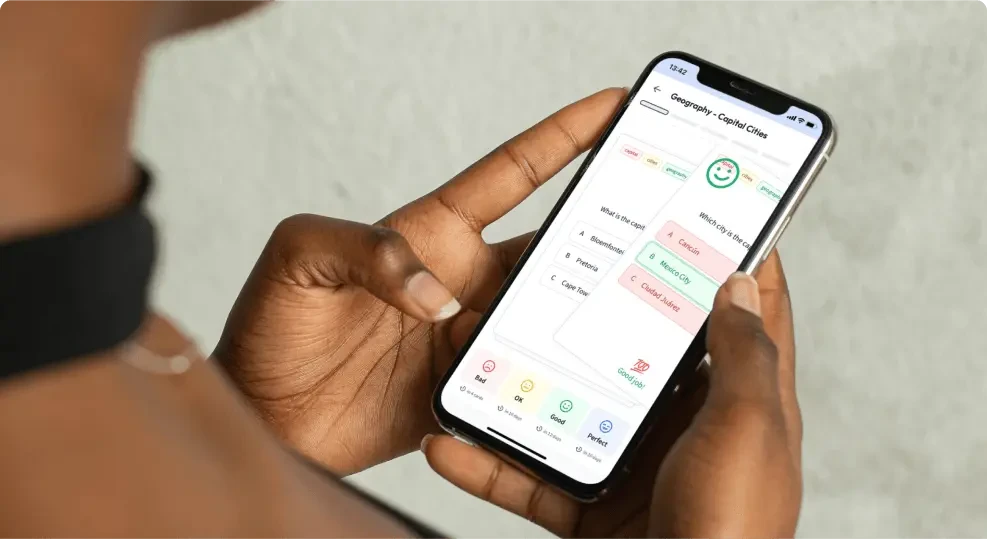
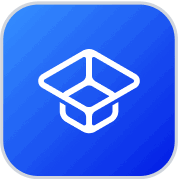
About StudySmarter
StudySmarter is a globally recognized educational technology company, offering a holistic learning platform designed for students of all ages and educational levels. Our platform provides learning support for a wide range of subjects, including STEM, Social Sciences, and Languages and also helps students to successfully master various tests and exams worldwide, such as GCSE, A Level, SAT, ACT, Abitur, and more. We offer an extensive library of learning materials, including interactive flashcards, comprehensive textbook solutions, and detailed explanations. The cutting-edge technology and tools we provide help students create their own learning materials. StudySmarter’s content is not only expert-verified but also regularly updated to ensure accuracy and relevance.
Learn more