Understanding the Concept: Define Inductive Effect in Organic Chemistry
In organic chemistry, the Inductive Effect refers to the distribution of electric charge along a chain of atoms in a molecule due to electronegativity difference or the polarisation of chemical bonds. This results in a chain of polarised bonds in a molecule and significantly influences the molecule's chemical reactivity.
The Basics of Inductive Effect
The Inductive Effect operates in a molecule where there are atoms or groups of varying electronegativities. Polarisation of bonds results from this difference and influences several chemical properties of the molecule. To understand the inductive effect, it helps to remember some key points:- The effect is transmitted through the chain of atoms in a molecule.
- It decreases as you move further from the source of polarisation.
- A stronger electronegativity leads to a more pronounced inductive effect.
Chlorine being more electronegative tends to pull the shared pair of electrons closer to itself, leading to a dipole moment in the molecule and a partial negative charge on the Chlorine atom and a partial positive charge on the Hydrogen atom. Here, Chlorine is said to be electron-withdrawing, and this effect of pulling electrons away from Hydrogen through the bond is the inductive effect.
Identifying the Importance of Inductive Effect in Molecular Structures
The inductive effect is crucial in determining the behaviour of molecules in various chemical reactions. It impacts molecular properties like acidity and basicity, reactivity, and the stability of reaction intermediates. Understanding the inductive effect is fundamental in organic chemistry, particularly in:- Visualising how reactions occur
- Predicting the products of reactions
- Determining the stability of molecules or intermediates in a reaction
In the reaction, acetic acid donates a proton (H+) to water. The proton is removed from the -COOH group, leaving a carboxylate ion (\(CH_3COO^-\)). The electron-withdrawing inductive effect of the carbonyl group (C=O) contributes to the stability of the carboxylate ion by dispersing the negative charge, making acetic acid acidic.
Hence, the inductive effect not only influences the distribution of charge in a molecule but also significantly impact how a molecule behaves in a chemical reaction - particularly acid-base reactions and reactions involving polar mechanisms. Understanding the inductive effect is integral to creating a foundation in organic chemistry and to advance in studying more complex chemical reactions.
Exploring the Positive Inductive Effect in Organic Chemistry
In organic chemistry, the inductive effect isn't solely a single, overarching concept. It branches into two categories: positive and negative. In this section, you'll delve into the positive inductive effect—defining it, identifying its manifestations, and recognising its critical role in various organic reactions.What is Positive Inductive Effect?
The Positive Inductive Effect (+I effect) occurs when atoms or groups of atoms, while being part of a molecule, have a tendency to push or donate electrons towards other atoms or groups within the same molecule. These electron-donating groups or atoms are less electronegative, and they allow electrons to flow towards other atoms or groups.
- The effect is a result of the weaker electronegativity of an atom or group of atoms.
- It enables electron dispersion and distribution throughout the molecule.
- Ethyl (CH3-), Methyl (CH2-), and other alkyl groups commonly exhibit the +I effect.
In ethane (\(CH_{3}CH_{3}\)), the ethyl group donates electrons towards the other carbon atom due to its lesser electronegativity. The electron-donating characteristic of the ethyl group highlights the positive inductive effect.
Manifestations of Positive Inductive Effect
The manifestations of the +I effect extend beyond the simplistic electron donating; it directly influences the stability and reactivity of different molecular structures. A notable manifestation is the stabilisation of carbocations. Carbocations are ions with a positively-charged carbon atom. In the presence of electron-donating groups (+I effect), the positive charge on the carbocation gets delocalised or spread out, stabilising the ion. Consider the stability of the following carbocations:Ethyl carbocation (\(CH_{3}CH_{2}^{+}\)) | > | Methyl carbocation (\(CH_{3}^{+}\)) |
The ethyl carbocation is more stable than a methyl carbocation because of its additional ethyl group. The ethyl group donates electrons towards the positively charged carbon atom, effectively delocalising the positive charge and stabilising the carbocation. This stabilisation is a direct manifestation of the positive inductive effect.
Role of Positive Inductive Effect in Organic Reactions
The +I effect plays a vital role in predicting the outcomes of various organic reactions, especially in dictating the stability of reaction intermediates and influencing reaction mechanisms. One crucial arena where the +I effect significantly influences the outcome is nucleophilic substitution reactions. Substitution reactions occur when an atom or group in a molecule gets replaced by another atom or group, often involving a nucleophile (electron-rich species) and a substrate (electron-deficient species). In nucleophilic substitution reactions, substrates that are more positively polarised or activated towards nucleophilic attack (due to the +I effect) often react faster. Thus, understanding the +I effect helps predict the reactivity of substrates in these reactions. Another area where the +I effect holds relevance is in determining the acidity of organic acids. It impacts the ionisation of acid molecules and subsequently their acid strength. Consider the acidic strength of the following carboxylic acids:Chloroacetic acid (\(ClCH_{2}COOH\)) | < | Acetic acid (\(CH_{3}COOH\)) |
Chloroacetic acid is less acidic than acetic acid. The chlorine atom in chloroacetic acid, being highly electronegative, withdraws electrons and destabilises the resultant anion, rendering the acid less likely to donate a proton. On the contrary, the methyl group in acetic acid exhibits a +I effect, pushing electrons towards the carboxylate ion and thus stabilising it, rendering acetic acid more acidic.
Grasping the Essentialities of Negative Inductive Effect
Delving into the intricacies of the Inductive Effect, there's another subset of the effect to understand: the Negative Inductive Effect. Similar to the Positive Inductive Effect, it operates within a molecular system, modulating the distribution of electrons and further influencing the system's chemical properties.Gaining Insight into the Negative Inductive Effect
The Negative Inductive Effect (-I effect) characterises atoms or groups of atoms that tend to pull or accept electrons from other atoms or groups within the same molecule. These electron-withdrawing groups or atoms are generally more electronegative, exerting an influence on the adjoining bonds to move electrons in their direction.
- The effect arises due to the enhanced electronegativity of an atom or group.
- It enhances electron carrying towards the electron-hungry atom or group.
- Nitro (\(NO_{2}\)), Carbonyl (\(C=O\)), and Halogens (F, Cl, Br, I) are common groups showing the -I effect.
In the molecule of Chloromethane (\(CH_{3}Cl\)), the Chlorine atom with higher electronegativity pulls the shared pair of electrons closer to itself, causing a polarisation of the bond. Hence, exhibiting the negative inductive effect.
Explaining Carbocation Inductive Effect
The Negative Inductive Effect plays a significant role in stabilising carbocations—ions bearing a positive charge on the carbon atom. Carbocations are electron-deficient species. Introducing an electron-withdrawing group showing a -I effect near a carbocation helps distribute the positive charge, thereby stabilising the ion. Consider an example with a carbocation next to a nitro group which is a -I effect exhibitor:In a molecule of nitromethane (\(CH_{3}NO_{2}^{+}\)), the nitro group, displaying the -I effect, withdraws electrons from the adjacent carbon atom. This electron movement helps dilute the positive charge on the carbocation, thus contributing to its stability.
Application of Negative Inductive Effect in Organic Compounds
The -I effect carries a significant influence across various reactions in organic chemistry. It helps ascertain the acidity or basicity of organic compounds, dictate the strength of nucleophiles and electrophiles, and determine reaction mechanisms. In the context of acid-base reactions, more the -I effect from electron-withdrawing groups, stronger the acid, as the phenomenon of electron-withdrawal helps stabilise the resultant conjugate base. Consider acidity comparison of acetic acid and fluoroacetic acid, for instance:Fluoroacetic acid (\(FCOOH\)) | > | Acetic acid (\(CH_{3}COOH\)) |
Fluoroacetic acid is more acidic than acetic acid. The electronegative fluorine atom exhibits a powerful -I effect in fluoroacetic acid. This withdrawal of electrons from the carboxylate ion stabilises it, making the acid more likely to donate a proton and display increased acidity. By contrast, acetic acid, with a +I effect exhibiting methyl group, is less capable of stabilising its carboxylate ion, making it less acidic.
The Inductive Effect Examples in Practical Applications
Deep in the trenches of organic chemistry, both positive and negative inductive effects find extensive applications, influencing molecular properties and dictating the course of various chemical reactions. These effects are not just confined to the theoretical realm but surface in myriad practical applications, from influencing medicine to modifying polymers. Real-life examples are certainly no mere abstraction; rather, they provide a tangible context making these effects more comprehensible and relatable.Insightful Examples of Negative and Positive Inductive Effects
The influence of inductive effects, both positive and negative, dwells within the very warps and wefts of organic chemistry. These effects, being pervasive, manifest in the simplest to the most complex of molecular systems. Identifying the prevalence of these effects in real-life situations is decoder-key to understanding their role in organic chemistry. The negative inductive effect, for instance, plays a significant role in modifying the acidic or basic properties of molecules. One such example slithers in the very ordinary vinegar you use in the kitchen—acetic acid (\(CH_{3}COOH\)). In acetic acid, the electron-withdrawing power of the carbonyl group (\(C=O\)) through the -I effect enhances the acidity of the molecule. By stabilising the conjugate base (\(CH_{3}COO^{-}\)) through electron withdrawal, the carbonyl group makes the acid more likely to donate its proton, thereby amplifying its acidic strength. A similar player is fluoroacetic acid (\(FCOOH\)), appearing in various pest control products. The fluorine atom, showing a robust -I effect, enriches the acidity of this molecule. With its high electronegativity, it draws electrons from the carboxylate ion to stabilise it, boosting the tendency of the molecule to lose a proton. Positive inductive effect too has a story to tell, majorly influencing the stability of carbocations. A glance at drugs containing phenylephrine, used as a nasal decongestant, presents an excellent example. Phenylephrine's complex structure contains an ethyl group, which, by virtue of the +I effect, donates electrons to the carbocation present in its molecular structure, thereby adding to its stability. This stability is vital for the efficacy of the drug, making it more resistant to metabolic degradation in the body. Another example of the +I effect surfaces in the everyday plastic—polyethylene. The dominance of the +I effect in polyethylene’s molecular structure from the extensive presence of only hydrogen and carbon creates a high degree of electron cloud density. This richness results in a material that is highly resistive to electricity!Real Life Instances of Inductive Effect in Chemistry
In the grand tapestry of chemistry, the inductive effect leaves its prints in abundant places. It plays into several chemical phenomena around you, from the tanginess of your food, the efficacy of the medicine you take, the strength of the plastic you use, to the smell of the perfume you wear—the inductive effect cloves a role everywhere. Take, for example, the basicity of amines in compounds. The increased electron density on nitrogen due to the +I effect from neighbouring alkyl groups boosts the basicity of amines. For instance, in medicines like chlorpheniramine (antihistamine), the presence of alkyl group exhibits a +I effect, increasing the electron density of amine, thereby enhancing its basicity. The world of polymers also showcases plenty of examples. High-density polyethylene (HDPE) used in making bottles, containers, etc., comprises a long-chain of alkyl groups. They manifest a +I effect, making the material an electrical insulator. This effect is also why the material is resistant to many solvents. A rather delightful example of inductive effect surfaces in the realm of perfumery. Terpenes, organic compounds that constitute the primary component in essential oils used in perfumes, owe their distinctive scent to the configuration of their molecular structure. The inductive effects from various functional groups present in the terpenes affect the concentration of their aroma and influence their smell. The inductive effect is thus etched deeply into the chemical overlay of the world around you. Recognising its presence in such various examples in real life renders an in-depth understanding of this concept, creating a bridge between theory and practice in the world of organic chemistry.Distinguishing Between the Inductive and Mesomeric Effect
A profound understanding of organic chemistry entails discerning the various effects that govern the behaviour of atoms and molecules. Two such important effects are the Inductive Effect and the Mesomeric Effect. While both dictate the distribution of electron density within a molecule, they are distinct in their influence, mechanisms, and instances of application.Defining the Difference Between Inductive and Mesomeric Effect
The Inductive Effect pertains to the displacement of electrons along a saturated carbon chain due to the electronegativity difference between atoms. This electron displacement can be either towards a more electronegative atom (Negative Inductive Effect, -I) or away from a less electronegative atom (Positive Inductive Effect, +I).
- The Inductive Effect operates in a chain of σ bonds, whereas the Mesomeric Effect acts within π bonds and lone pairs.
- The Inductive Effect causes a permanent polarisation of the bond, while the Mesomeric Effect leads to the creation of resonating structures.
- The Inductive Effect declines with distance, disappearing after three to four bonds, while the Mesomeric Effect extends across the entire conjugated system.
- The Inductive Effect is a two-centre effect, impacting just adjacent bonds, whereas the Mesomeric Effect involves the whole molecule, making it a multi-centred effect.
Highlighting the Unique Attributes of Inductive and Mesomeric Effect in Organic Chemistry
Beyond these contrastive points, the unique attributes of both effects lend them distinct roles within the realms of organic chemistry. The inductive effect, for instance, is instrumental in determining the acidity or basicity of organic compounds. By influencing the polarity of bonds and thereby the concentration of charge at particular regions of a molecule, the Inductive Effect categorically influences the acidic and basic tendencies of the molecule. Consider molecule of acetic acid (\(CH_{3}COOH\)). Here, the electron-withdrawing carbonyl group (\(C=O\)) shows a -I effect, thereby enhancing the acidity of the molecule by aiding in the stabilisation of the resulting carboxylate (\(CH_{3}COO^{-}\)) ion. The Mesomeric Effect, on the other hand, plays a significant role in lending stability to molecules with π bonds and lone pairs of electrons. By distributing the electron cloud over a broader region, it distributes the associated charge more evenly, thereby stabilising molecules. For example, take a look at ozone (\(O_{3}\)). In ozone, the π electrons of the molecule are delocalised over all three oxygen atoms. This delocalisation of electrons leads to two resonating structures of ozone as a result of the Mesomeric Effect, enhancing the molecule's stability. The ultimate role these effects play within organic chemistry is modulating molecular behaviour based on the distribution of electron density. Once understood in their respective contexts, the inductive and mesomeric effects can become powerful conceptual tools—allowing you to predict chemical reactions, understand molecular dynamics, and fathom the complexities of organic chemistry with a greater comprehension.Inductive Effect - Key takeaways
- Inductive Effect: A key concept in organic chemistry, referring to how electron density gets distributed across a molecule, significantly impacting its behaviour in chemical reactions.
- Positive Inductive Effect: Occurs when atoms or groups in a molecule, being less electronegative, donate electrons towards other atoms or groups within the same molecule. This effect tends to stabilise carbocations by dispersing the positive charge.
- Negative Inductive Effect: Characterises atoms or groups in a molecule that pull or accept electrons from other atoms or groups due to their enhanced electronegativity. It helps stabilise carbocations and increase acidity of compounds.
- Inductive Effect Examples: The positive and negative inductive effects have applications in various areas, from influencing the acidity or basicity of compounds to dictating the course of chemical reactions, and can be seen in everything from everyday items (like vinegar) to drugs and polymers.
- Difference between Inductive and Mesomeric Effect: These are two different effects governing the behaviour of atoms and molecules in organic chemistry, with the inductive effect referring to the electron-pushing or pulling nature of atoms or groups, and the mesomeric effect related to the delocalisation of π electrons.
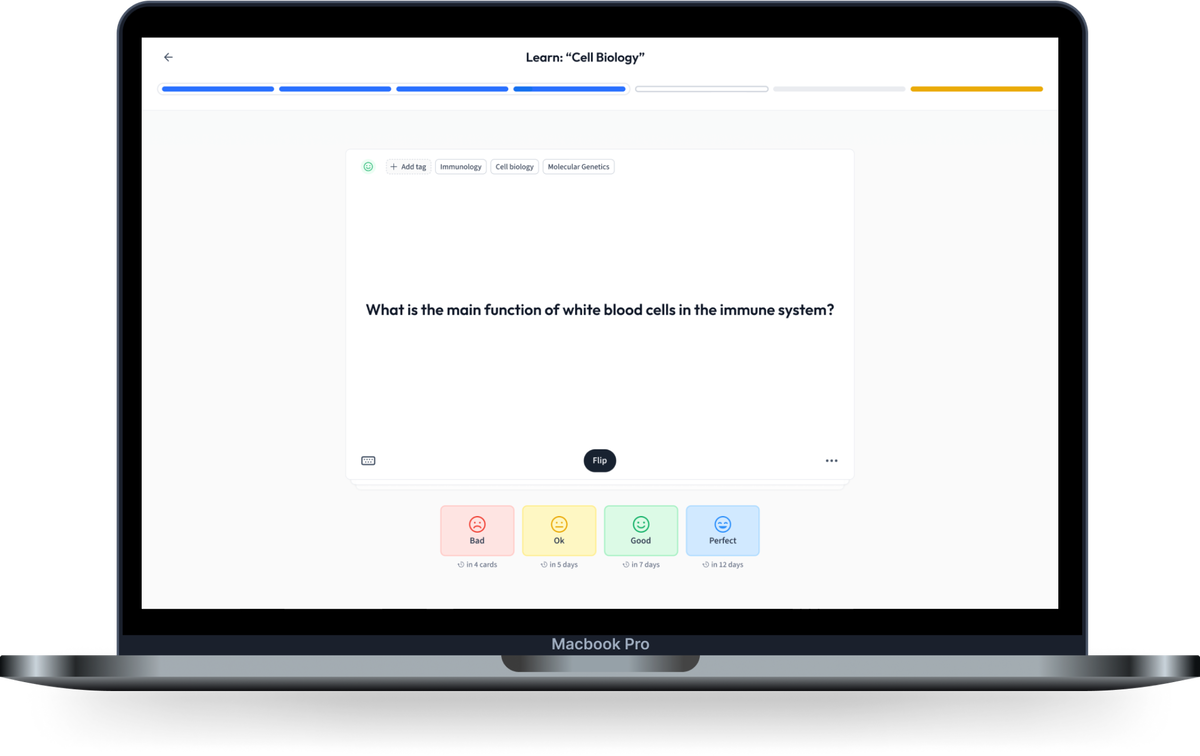
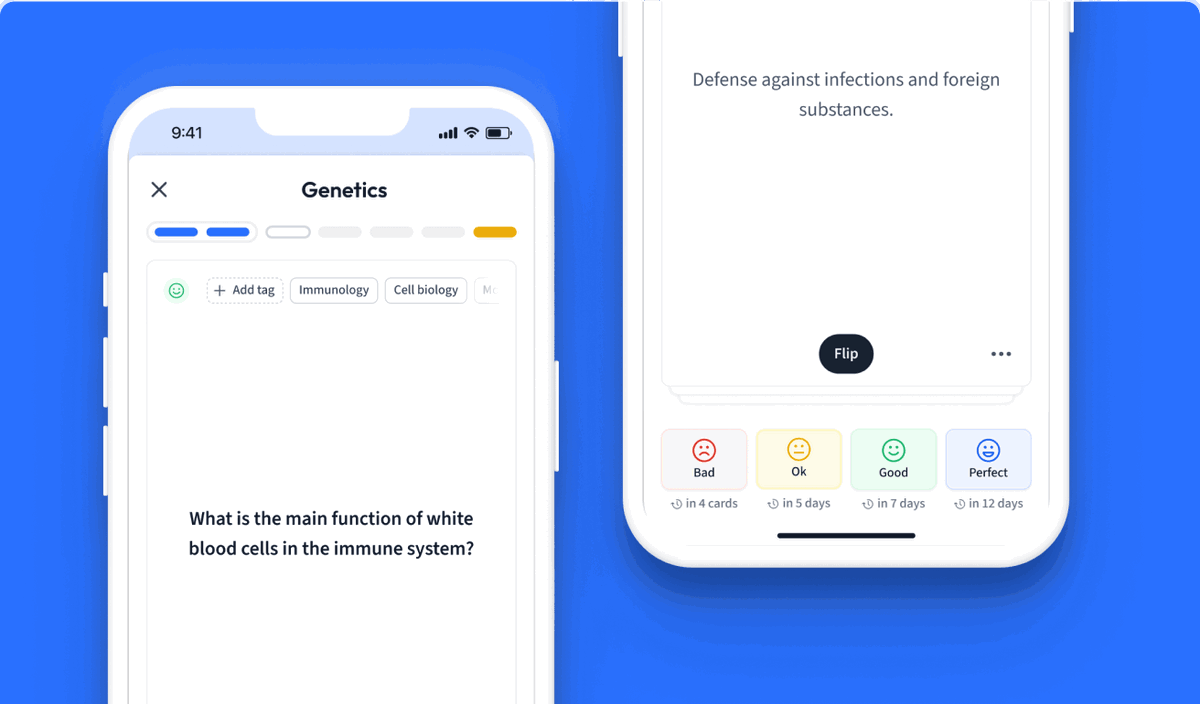
Learn with 15 Inductive Effect flashcards in the free StudySmarter app
We have 14,000 flashcards about Dynamic Landscapes.
Already have an account? Log in
Frequently Asked Questions about Inductive Effect
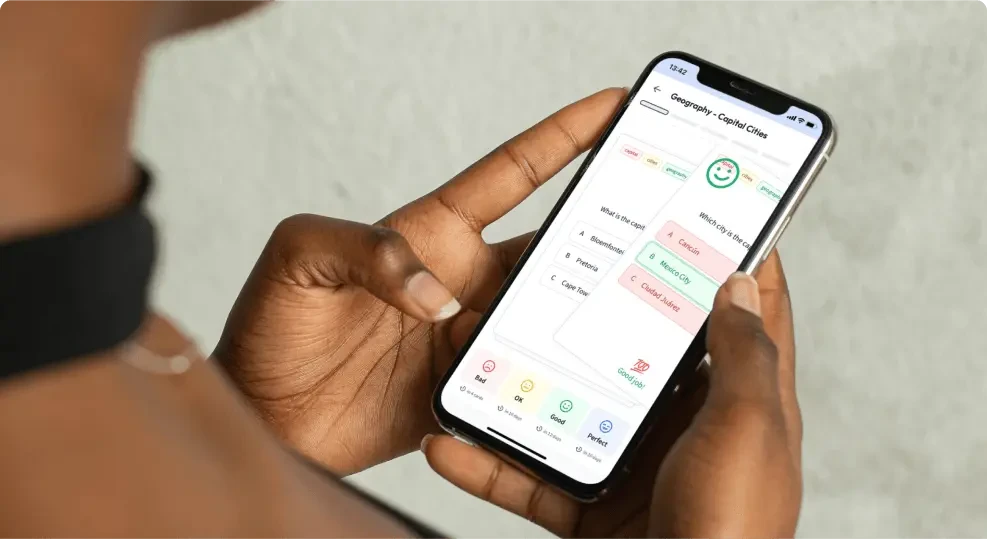
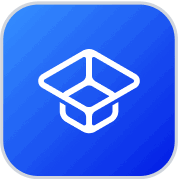
About StudySmarter
StudySmarter is a globally recognized educational technology company, offering a holistic learning platform designed for students of all ages and educational levels. Our platform provides learning support for a wide range of subjects, including STEM, Social Sciences, and Languages and also helps students to successfully master various tests and exams worldwide, such as GCSE, A Level, SAT, ACT, Abitur, and more. We offer an extensive library of learning materials, including interactive flashcards, comprehensive textbook solutions, and detailed explanations. The cutting-edge technology and tools we provide help students create their own learning materials. StudySmarter’s content is not only expert-verified but also regularly updated to ensure accuracy and relevance.
Learn more