Understanding Spin Orbit Coupling
Spin Orbit Coupling is a fascinating concept you'll encounter in quantum chemistry. Understanding this effect can truly elevate your comprehension of how atomic and molecular systems behave.An Introductory Look at Spin Orbit Coupling Chemistry
Before digging deep into Spin Orbit Coupling, it's crucial for you to recognize that chemistry isn't solely about experiments, but there's an intricate dance of particles on a quantum level that dictates every reaction you observe.In the world of quantum mechanics, Spin Orbit Coupling refers to the interaction of an electron's spin with its motion. To put it simply, it's the intertwining of an electron's inherent angular momentum (spin) and the angular momentum obtained from orbiting the nucleus.
Zeeman Effect: a well-documented instance where Spin Orbit Coupling plays a significant role. When a magnetic field is applied to an atom, the energy levels of electrons can split, leading to fascinating spectral line patterns.
The Basics of Spin Orbit Coupling Definition
Think of Spin Orbit Coupling as a sort of gateway that allows you to explore the consequences of quantum physics in your chemistry classroom. By comprehensibly defining this concept, you are essentially cracking the code that unravels the incredible world of quantum effects in atoms and molecules.
Spin Orbit Coupling Examples in Organic Chemistry
Exploring Spin Orbit Coupling in the context of organic chemistry can help elucidate how this concept comes to life in the chemical reactions you may perform in the lab.One common example of Spin Orbit Coupling at work in organic chemistry is intersystem crossing. Intersystem crossing is the process by which molecules transition between electronic states of different multiplicity. This quantum leap can provide a new route for molecules to dispose of extra energy, leading to various consequences in reaction outcomes.
Implications of Spin Orbit Coupling in Chemistry
As you delve into the implications of Spin Orbit Coupling, you might wonder, "why should I, as a chemistry student, bother about this?" First of all, let's address the elephant in the room - Spin Orbit Coupling is paramount in understanding the structure and properties of heavy elements. Without considering this coupling, it's practically impossible to interpret the electronic structures of compounds containing heavier elements accurately.For instance, Spin Orbit Coupling plays a critical role in interpreting the unusual colour of gold and the low melting point of mercury. In both scenarios, the understanding of these peculiarities lies in Spin Orbit Coupling, emphasising its integral role in these phenomena.
Detailed Calculation of Spin Orbit Coupling Constant
In the fascinating world of quantum mechanics, every theory, every phenomenon comes down to calculations that describe how particles interact. One such fundamental calculation is the Spin Orbit Coupling constant, a calculation that's critical for a cogent understanding of atom and molecule dynamics.How to Calculate Spin Orbit Coupling Constant: A Complete Guide
Visualise the Spin Orbit Coupling constant as a numerical value representing the strength of interaction between the electron's spin and its orbital motion. This interaction leads to shifts or splittings in energy levels significantly impacting the chemical and physical properties of an atom. How does one calculate this constant, one might wonder? As described earlier, the Spin Orbit Hamiltonian states: \[ H_{SO} = \xi(r) \cdot \boldsymbol{L} \cdot \boldsymbol{S} \] Where \(\xi(r)\) is the Spin Orbit Coupling constant. This expression is not a 'one-size-fits-all' calculation. It depends on the electron's radial distance from the nucleus (\(r\)), and so it varies accordingly.Step-by-Step Calculation of Spin Orbit Coupling Constant
Here is a streamlined method to calculate the Spin Orbit Coupling constant:- Start with the atomic number (\(Z\)) of the atom in question. This information can easily be gathered from the periodic table.
- Figure out the principal quantum number (\(n\)) as well as the azimuthal quantum number (\(l\)). These details are determined by the electron's location in an atom, its energy level and orbital shape respectively.
- Establish the radial distance of an electron from the nucleus (\(r\)). Remember, the further out an electron, the weaker the Spin Orbit Coupling.
- Make use of the Spin Orbit Coupling constant equation: \[ \xi(r) = \frac{\hbar^{2}}{2m^{2}c^{2}} \cdot \frac{1}{r^{3}} \cdot \frac{dV(r)}{dr} \] Here, \(\hbar\) is the reduced Planck constant, \(m\) is the electron mass, \(c\) is the speed of light, \(V(r)\) is the potential and \(dV(r)/dr\) is the derivative of this potential with respect to \(r\).
- Bear in mind that this equation is purely quantum mechanical and epitomises the coupling constant's dependence on the electron's radial behaviour in an atom.
Factors Impacting the Calculation of Spin Orbit Coupling Constant
Factors effecting the calculation of Spin Orbit Coupling constant predominantly include the quantum numbers and the electron's radial distance from the nucleus. However, there's more to it. The charge of the nucleus (atomic number), the speed of the electron and the potential (\(V(r)\)) in which the electron is moving, also fundamentally impacts this calculation. The Spin Orbit Coupling constant would be different for an electron in a \(1s\) orbital compared to one in a \(2p\) orbital. This is due to the differing radial behaviour of these orbitals. Furthermore, in multi-electron atoms, electron-electron interactions play a part in Spin Orbit Coupling, thus rendering its calculation quite complex. Keep in mind that the Spin Orbit Coupling constant is not always easily calculated. For complex systems like molecules, it needs to be computed using robust computational methods such as Hartree-Fock or Density Functional Theory, widely employed in computational chemistry. By understanding the factors affecting the calculation, you can approach the Spin Orbit Coupling constant with comprehensive understanding, detailing how this complex yet intriguing constant is so pivotal in the understanding of the elements that constitute our universe.Practical Application and Examples of Spin Orbit Coupling
In the multifaceted domain of chemistry, Spin Orbit Coupling is far from being a theoretical abstraction. Its tentacles spread far and wide, impacting all spheres from atomic structure understanding to spectral analysis and chemical reactivity determination. Grasping this concept threads the needle for interpreting several chemical phenomena in an unanticipated light.Real-life Spin Orbit Coupling Applications in Chemistry
Chemistry is not just a spectator to the enthralling dance of particles but an active participant too. And, in this elaborate ballet of electrons and nuclei, Spin Orbit Coupling is the choreographer, dictating every twirl and sway. That is precisely why you encounter its applications ubiquitously in the chemical realm. One of the pinnacle applications of Spin Orbit Coupling is the apt explanation of atomic spectra. As you know, atomic spectra are the fingerprints of each element, as each one imparts its unique spectral pattern. But did you know that these spectral lines, fascinating in their complexity, are intimately influenced by Spin Orbit Coupling? In the realm of spectroscopy, especially X-ray or Auger spectroscopy, Spin Orbit Coupling cannot be overlooked. Here, the energy splittings generated by the coupling play a pivotal role in determining spectral intensities. This allows for meticulous analysis and identification of heavier elements where the effect is pronounced. Another spectacular manifestation of Spin Orbit Coupling is in the phenomenon known as the 'Zeeman Effect', where external magnetic fields cause splitting of spectral lines. Each split line correlates to a different transition between energy states, altered by Spin Orbit Coupling. Moreover, Spin Orbit Coupling impacts chemical reactions. It orchestrates a phenomenon called 'intersystem crossing' frequently seen in photochemistry. This occurs when a molecule hops between two electronic states that have different spin multiplicities. Surprisingly, such transitions can even be possible despite being 'spin forbidden' due to the intervention of Spin Orbit Coupling.Lab Examples of Spin Orbit Coupling Application
Lab scenarios provide a phenomenal platform for observing Spin Orbit Coupling in action. If you've experienced the excitement of witnessing a spectral line split in a magnetic field, or watched a 'spin-forbidden' reaction unfolds right before your eyes, you've seen the magic of Spin Orbit Coupling in real-time. Among the many examples that stand out, the colorimetric analysis of elements, particularly when dealing with heavy metals like gold or mercury, is a much-cited one. It allows the Spin Orbit Coupling effect to be clearly observed. Additionally, catalytic reactions, especially those involving transition metal complexes, illustrate complex spin states. In many such reactions, Spin Orbit Coupling can be the pivot that dominates the reaction mechanism. One stellar instance is the stoichiometric reaction steps in classic homogeneous catalytic cycles, where intersystem crossing may provide an alternate reaction pathway.Interpreting Spin Orbit Coupling: Examples and Analysis
Interpreting Spin Orbit Coupling resides in understanding how it influences and shapes observations. For students performing experiments and observations in the lab, it's crucial to make connections between theory and practice and appreciate the role of quantum phenomena in perceptible outcomes. You can glean insights into Spin Orbit Coupling from the elementary flame test. If students look more closely at the 'flickering' colours they observe when a metal salt is introduced into a flame, they might just recognise the result of differing energy states due to Spin Orbit Coupling. Even in the computational simulation of elemental properties or molecular behaviours, acknowledging Spin Orbit Coupling can enrich one's interpretation of results. An excellent instance is the calculation of magnetic susceptibility. With the knowledge that Spin Orbit Coupling factors in, you can understand why atomic magnetism isn't just about d-orbitals.Causes of Spin Orbit Coupling: An In-depth Explanation
Spin Orbit Coupling is born out of the fundamental constructs of quantum mechanics. To be specific, it is a quantum-mechanical effect that arises from the interaction between the electron's spin and its motion about the nucleus. The roots of Spin Orbit Coupling may be traced back to two essential features of electron dynamics—firstly, the electron spin, the intrinsic angular momentum of the electron. Secondly, to the orbital motion of the electron around the nucleus. Imagine the two as interconnected gears in a clock that perpetually interact—the quantum mechanical 'dance' in every atom. Crucially, the shape and behaviour of this 'dance' is not uniform across different electrons. It varies depending on the orbital an electron resides in. The transition from spherical (\(s\)) to dumbbell-shaped (\(p\)) orbitals to more complex shapes (\(d\) and \(f\)), signifies varying extents of the electron's angular momentum. This, combined with the electron's spin, creates the choreography for the Spin Orbit Coupling. The crucial aspect that underlies Spin Orbit Coupling is relativistic effects. As an electron zips around the nucleus at speeds comparable to the speed of light, relativistic adjustments seep into the picture. Consequently, the electron senses the nucleus's electric field in a skewed manner, leading to a magnetic interaction with the electron's spin—thereby giving rise to Spin Orbit Coupling. Lastly, the electron's radial distance, or how far from the nucleus it is whirling, also affects the extent of Spin Orbit Coupling. As you might guess, electrons further away in larger orbitals interact less significantly with the nucleus and hence, experience weaker Spin Orbit Coupling. So, in essence, it is these atomic subtleties and relativistic mechanics that define the cause and occurrence of Spin Orbit Coupling.The Role of Spin Orbit Coupling in Chemical Reactions
While at first glance, it might seem that quantum phenomena like Spin Orbit Coupling pertains to the realm of atomic physics rather than chemical reactions, this presumption is far from accurate. Dig a little deeper, and you'll find that the concept is ingrained in our understanding of chemical processes, particularly reactions involving heavier elements and certain specific cases in organic chemistry.Understanding the Effect of Spin Orbit Coupling in Organic Chemistry
The domain of organic chemistry, laden with a rich variety of molecules and countless reaction mechanisms, offers a fertile ground for observing the effects of Spin Orbit Coupling. This quantum mechanical phenomenon, while relevant to all, is especially pronounced in heavy atoms and is pivotal in particular reactions and pathways within the arena of organic chemistry. One prime example of Spin Orbit Coupling's influence on organic chemistry surrounds halogen-containing organic compounds. These molecules, which often involve heavy halogens like bromine or iodine, showcase significant Spin Orbit Coupling due to the weighty nature of these atoms. This effect can lead to unique photophysical properties, which forms the foundation of a variety of luminescent organic materials. In such compounds, the heavy atoms give rise to a significant Spin Orbit Coupling effect that can, for instance, facilitate transitions to 'forbidden' triplet states. A typical example is the Photochemical Reactions involving converting 'singlet' states to 'triplet' states, referred to as 'intersystem crossing'. The facilitation of such transitions via Spin Orbit Coupling, thanks to the heavy atoms, promulgates unique pathways, leading to products that would otherwise be inaccessible.Intersystem Crossing: A radiationless process, governed by the Spin Orbit Coupling, whereby a molecule moves between states of different spin multiplicity (most commonly from a singlet to a triplet state, or vice versa).
The Chain Reaction: How Spin Orbit Coupling Influences Chemical Processes
Elucidating how exactly Spin Orbit Coupling influences chemical processes involves delving into the theory of reaction mechanisms. From a ground-level perspective, the theory of chemical reactivity and reaction rates hinge on the concept of potential energy surfaces, valleys and peaks representing various molecular states, and transition states, respectively. Now, this is where Spin Orbit Coupling joins the spectacle.Potential Energy Surfaces: A theoretical construct that allows visualising the energy of a system (often of molecules during a chemical reaction) with respect to its geometric configuration or composition.
Advancing your Knowledge on Spin Orbit Coupling
Spin Orbit Coupling, an aspect heavily rooted in Quantum Mechanics, is no walk in the park when it comes to fully grasping the concept. However, fear not, as there is a wealth of resources for understanding and excelling in this subject matter. To completely fathom and appreciate the role of Spin Orbit Coupling in chemical reactions, a careful exploration of these resources is vital.Crucial Resources for Mastering Spin Orbit Coupling
For delving into the depths of Spin Orbit Coupling and understanding its effect on chemical reactions, various resources are at your disposal. Be it textbooks shedding light on the theory or online resources offering engaging content in an easily digestable format, you will find a plethora of options available.- Textbooks: Traditional textbooks still hold prominence when it comes to understanding complex quantum mechanical concepts like Spin Orbit Coupling. Books like "Quantum Mechanics and Path Integrals" by Richard P. Feynman and Albert R. Hibbs, "Quantum Mechanics: Concepts and Applications" by Nouredine Zettili, and "Modern Quantum Mechanics" by J.J. Sakurai elaborately explain Spin Orbit Coupling and its implications.
- Research papers: To grasp state-of-the-art applications of Spin Orbit Coupling and to understand how it shapes modern chemical research, digging into scientific literature can be of immense aid. Papers published in well-regarded scientific journals like 'Nature', 'Science', and 'Journal of the American Chemical Society' often flaunt the use of Spin Orbit Coupling in determining reaction rates or explaining unusual chemical behaviour.
- Online lectures: With the advent of digital learning platforms, a multitude of online lectures and courses are readily available to help unravel the mysteries of Quantum Mechanics and Spin Orbit Coupling. Websites like Coursera, Khan Academy, and edX offer a variety of courses tailored for different levels of learners.
Hamiltonian: In quantum mechanics, a Hamiltonian is a function that describes the total energy of a system. The Hamiltonian operator, \( \hat{H} \), used in the Schrödinger equation, represents the sum of kinetic and potential energies for all particles in the system.
Enhance your Understanding of Spin Orbit Coupling with these Top Methods
To truly comprehend Spin Orbit Coupling and its impact on chemical reactions, it's crucial to approach your learning from multiple angles. Here are some methods you can use:Method | Resources |
Solving Problems | Consistently doing hands-on problems from textbooks such as "Problems and Solutions on Quantum Mechanics" by Yung Kuo Lim or using problem-solving platforms like Chegg. |
Research-based learning | Engaging with cutting-edge chemical research that leverages Spin Orbit Coupling in significant ways. Use databases like PubMed and Google Scholar to uncover the most recent findings in your area of interest. |
Spin Orbit Coupling - Key takeaways
- Spin Orbit Coupling is an interaction between an electron's orbital motion and its spin, leading to shifts in energy levels that have a significant impact on an atom's physical and chemical properties.
- The Spin Orbit Coupling constant, which represents the strength of Spin Orbit Coupling, can be calculated by considering factors like the electron's radial distance from the nucleus, the atomic number, the electronic quantum numbers, and the potential in which the electron is moving.
- Spin Orbit Coupling is more than a theoretical concept and has practical applications in fields such as spectroscopy, magnetism, and reaction mechanisms. It can impact atomic spectra, cause magnetic splitting of energy levels (as seen in the Zeeman Effect) and influence intersystem crossing in photochemistry.
- The presence of Spin Orbit Coupling can be observed in experiments like colorimetric analysis of heavy metal elements and in computational simulation of atomic and molecular properties.
- The cause of Spin Orbit Coupling can be traced back to the two primary features of electron dynamics: the electron's spin and its orbital motion. It is also influenced by relativistic effects and the electron's radial distance from the nucleus.
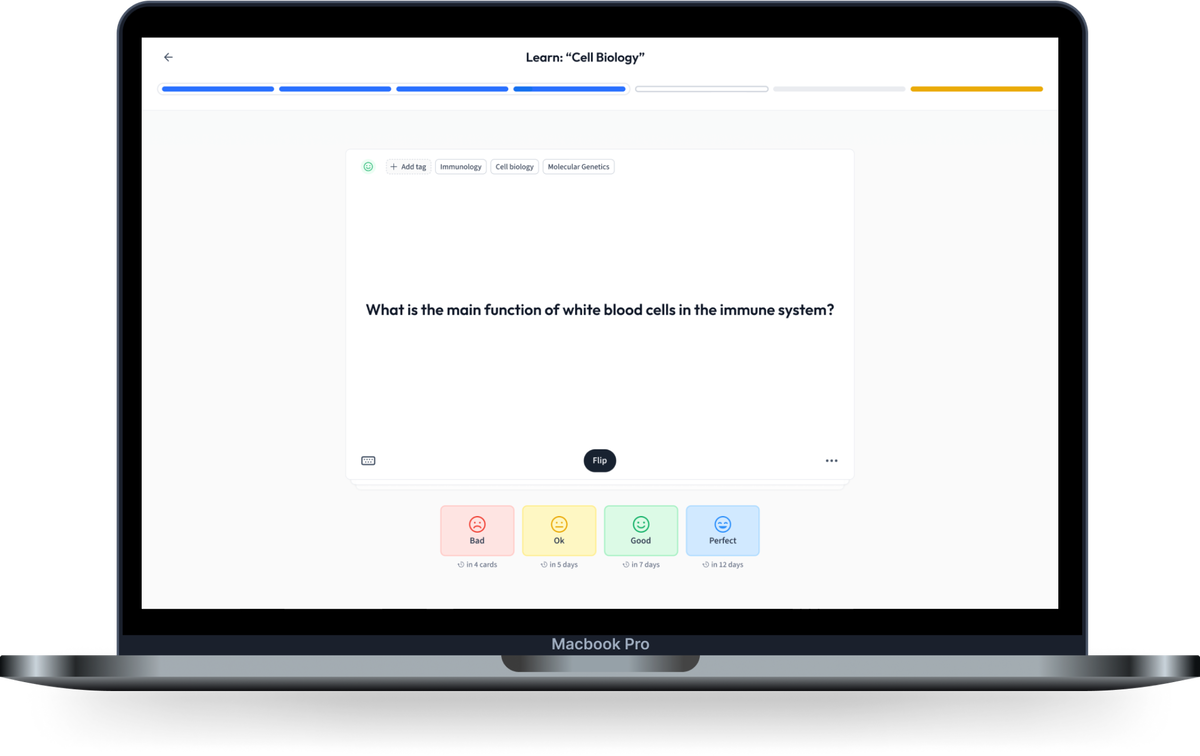
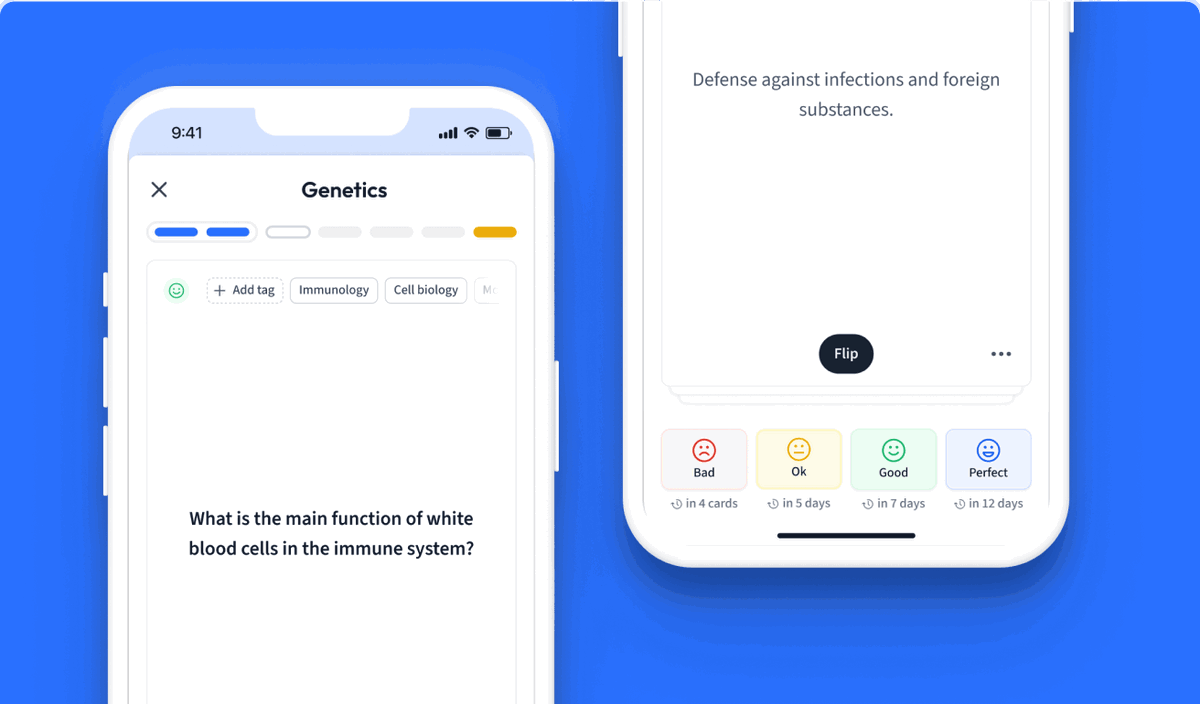
Learn with 15 Spin Orbit Coupling flashcards in the free StudySmarter app
We have 14,000 flashcards about Dynamic Landscapes.
Already have an account? Log in
Frequently Asked Questions about Spin Orbit Coupling
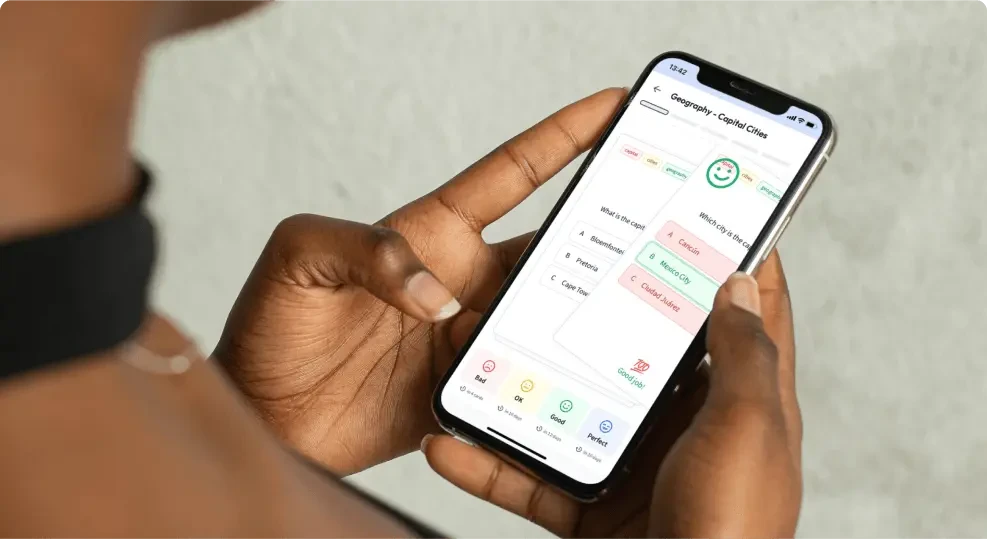
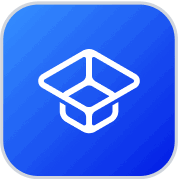
About StudySmarter
StudySmarter is a globally recognized educational technology company, offering a holistic learning platform designed for students of all ages and educational levels. Our platform provides learning support for a wide range of subjects, including STEM, Social Sciences, and Languages and also helps students to successfully master various tests and exams worldwide, such as GCSE, A Level, SAT, ACT, Abitur, and more. We offer an extensive library of learning materials, including interactive flashcards, comprehensive textbook solutions, and detailed explanations. The cutting-edge technology and tools we provide help students create their own learning materials. StudySmarter’s content is not only expert-verified but also regularly updated to ensure accuracy and relevance.
Learn more