Jump to a key chapter
Understanding the Oxidation of Alkanes Meaning
The oxidation of alkanes refers to a series of chemical reactions where alkanes, a hydrocarbon with the general formula \(C_nH_{2n+2}\), combine with oxygen in the air to form carbon dioxide and water, releasing energy in the process.
The Chemistry Behind the Oxidation of Alkanes
In essence, the oxidation reaction of alkanes is a combustion reaction. Combustion is a rapid oxidation process accompanied by the release of energy, which often manifests as heat or light or both. For instance, the general combustion reaction of an alkane with the simplest chemical structure, methane, is as follows: \[ CH_4 + 2O_2 \rightarrow CO_2 + 2H_2O \] In this reaction:- \(CH_4\) represents methane.
- \(O_2\) is the molecular oxygen from the air.
- \(CO_2\) is carbon dioxide produced.
- \(H_2O\) is the water generated.
In detail, the combustion reaction happens in two stages. Initially, the alkane reacts with oxygen to produce a series of complex intermediate hydrocarbons. These intermediates then further react with oxygen to form carbon dioxide and water, highest possible oxidation states of carbon and hydrogen, respectively.
Types of Reactions during the Oxidation of Alkanes
Different types of reactions may occur during the oxidation of alkanes, including:- Complete Combustion
- Incomplete Combustion
- Autooxidation
Factors Impacting the Oxidation of Alkanes
Several factors can impact the oxidation rate of alkanes:Structure of Alkane | The chain length and branching of the alkane can influence the reaction rate. More branched alkanes tend to oxidize faster than linear ones. |
Temperature | The rate of oxidation increases with temperature. Higher temperatures lead to faster reactions. |
Oxygen Concentration | Higher concentrations of oxygen lead to more complete combustion. |
An example of the effect of structure on oxidation rate can be observed in the difference between n-butane and isobutane. Though both have the same molecular formula (C4H10), isobutane, having a more branched structure, oxidizes more readily than n-butane, which has a straight chain.
Practical Oxidation of Alkanes Examples
When it comes to the oxidation of alkanes, examples are incredibly useful for better understanding the process. The practical examples provided below highlight some of the most common situations where the oxidation of alkanes takes place and give you an insight into the varied impact of this process.Highlighting Key Oxidation of Alkanes Examples
A notable example of the oxidation of alkanes is the controlled combustion of methane in natural gas stoves. In this case, methane \(CH_4\) combines with oxygen in the air to produce carbon dioxide \(CO_2\) and water \(H_2O\). The reaction, when balanced, can be summarised as follows: \[ CH_4 + 2O_2 \rightarrow CO_2 + 2H_2O \] Finding examples of incomplete and autooxidation might seem challenging but they're also widespread in our everyday routines. For incomplete combustion, imagine a car engine running in a low-oxygen environment. It produces energy, but instead of the ideal products (\(CO_2\) and \(H_2O\)), carbon monoxide (\(CO\)) and soot (carbon particles) are created as well. Autooxidation, as a slower and non-heat producing process, often takes place in the bodies of living organisms where alkane chains in fatty acids are oxidised to produce energy, a process fundamental to aerobic life.
Understanding Examples through Step-by-Step Processes
To learn more about oxidation reactions, the below practical example will guide you through a step-by-step understanding of the oxidation of ethane (\(C_2H_6\)), a common alkane:- Begin with the basic balanced chemical equation: \(C_2H_6 + \frac{7}{2}O_2 \rightarrow 2CO_2 + 3H_2O\)
- Understand the role of each reactant: \(C_2H_6\) is the alkane being oxidised, and \(O_2\) is the oxygen present in the air which can react with ethane.
- Next, view the products formed: \(CO_2\) is carbon dioxide which is produced as part of the process and \(H_2O\) is water.
- Register the energy release: This process also releases energy, typically in the form of heat or light.
Implications of Different Outcomes in Oxidation of Alkanes Examples
Different types of oxidation reactions result in different outcomes, all of which involve energy release but vary in the extent to which alkane is oxidized and the byproducts formed. For instance, in complete combustion, alkane is fully oxidised to \(CO_2\) and \(H_2O\), which are harmless end products. This reaction releases the maximum potential energy from the alkane and is hence used for various heat and energy-demanding activities, from powering automobiles to cooking food. However, during incomplete combustion, a lack of sufficient oxygen results in partially oxidised products - \(CO\), a poisonous gas, and carbon, also known as soot. This scenario not only represents an inefficient use of fuel that delivers less than its potential energy but also gives rise to harmful emissions. On another note, during autooxidation of alkanes, complex partial-oxidation products like alcohols, ketones, and acids are formed. These products, while not energy-giving, serve crucial roles in different chemical and biological processes that make up life as we know it.Real-world Oxidation of Alkanes Applications
Investigating the oxidation of alkanes provides critical insights into a multitude of applications in everyday life, ranging from energy production to pollution control. By understanding the chemistry behind this reaction, one can grasp how this fundamental reaction drives many industrial processes and influences our environment.Industrial Applications of the Oxidation of Alkanes
An understanding of the oxidation of alkanes is crucial in numerous industrial reactions. One of the most common instances is found in the petrochemical industry, where the combustion of alkanes is used to generate heat and drive various processes such as refining and petrochemical synthesis. The reaction proceeds as such: \[ C_nH_{2n+2} + (n + \frac{n}{2})O_2 \rightarrow nCO_2 + (n+1)H_2O \] In the above reaction, \(C_nH_{2n+2}\) represents an alkane where \(n\) is the number of carbon atoms in the alkane. Another significant industrial application is in the manufacturing of polymers. Oxidation of alkanes through various methodologies can lead to the creation of alcohols, aldehydes, ketones, and carboxylic acids, which serve as essential building blocks in plastic and polymer industry. Here are some notable industrial applications:- Air heating for commercial and residential buildings with gas furnaces
- Industrial heat production, for example, steam generation for turbines
- Use in internal combustion engines for vehicles
- Intermediate product synthesis, such as ethylene, through steam cracking
Exploring the Role of Oxidation of Alkanes in Energy Production
Living in the 21st century, you probably know that the largest share of our energy comes from fossil fuels - a substantial proportion of which are alkanes. The oxidation of these alkanes forms the basis of modern energy production. Two of the main energy production methods centred around the oxidation of alkanes are internal combustion engines and power stations.An internal combustion engine (ICE) is a heat engine where the combustion of a fuel takes place with an oxidiser (usually air) inside a combustion chamber. In automobiles, ICEs burn gasoline, diesel, or natural gas, releasing energy to perform work.
Environmental Implications of Oxidation of Alkanes Applications
While the oxidation of alkanes offers substantial benefits, particularly in energy production, it also leads to significant challenges. The leading concern revolves around the emission of carbon dioxide gases, a potent greenhouse gas that contributes to climate change. When burnt in sufficient oxygen, alkanes produce \(CO_2\) and \(H_2O\). With the vast quantities of alkanes we burn to meet our energy needs, the amounts of \(CO_2\) released represent a significant proportion of global carbon emissions. These emissions trap heat from the Sun and contribute to the warming of Earth's climate. Another byproduct of alkane oxidation is carbon monoxide (CO), particularly under conditions of incomplete combustion owing to low oxygen availability. CO is a poisonous gas detrimental to human health and contributes to ground-level ozone formation— a constituent of urban smog. Employing efficient technologies that ensure complete combustion, thus minimising CO production, is a crucial means of limiting these impacts. Furthermore, any unburnt alkanes that are released can contribute to the formation of ground-level ozone and other air pollutants, exacerbating health issues and climate change. Taking all these aspects into account, it becomes clear why sustainable and renewable energy sources are being researched and developed. While alkanes and their oxidation reactions have served us well for over a century, the environmental implications of their use are forcing us to rethink our energy strategies.Exploring Catalytic Oxidation of Alkanes
In our exploration of the oxidation of alkanes, it's vital to highlight a particular technique that has gained prominence in the field of chemistry: the catalytic oxidation of alkanes. This method, as the name suggests, uses a catalyst to speed up the oxidation process, leading to a more efficient reaction and opening up new possibilities for the use and application of alkanes.Understanding the Catalytic Oxidation Process
At its core, catalytic oxidation of alkanes involves the addition of a catalyst to the reaction mixture to boost the rate of oxidation. In the absence of sufficient oxygen and high temperature, alkanes are relatively inert and resistant to oxidation, limiting their usefulness in areas such as energy production and chemical synthesis. The use of a catalyst can overcome this challenge by promoting the formation of reactive intermediary species, which can undergo oxidation more readily. Depending on the type and chemical nature of the selected catalyst, different reaction mechanisms are involved. For example: - In the process that uses transition metal catalysts (such as vanadium, molybdenum), the catalyst first undergoes reduction and then re-oxidation while oxidising the alkane. - In another process, known as the 'free radical mechanism', a radical initiator (often a peroxide) is used to generate alkyl radicals that can react more readily with oxygen. Here is a simple example: \[ \begin{tabular}{|c|c|} \hline \text{Step 1: Generation of Alkyl Radicals} & \(R-H + Init\longrightarrow R. + Init. - H\)\\ \hline \text{Step 2: Reaction with Oxygen} & \(R. + O_2 \longrightarrow ROO.\)\\ \hline \text{Step 3: Further Reaction} & \(ROO. + R-H \longrightarrow ROOH + R.\)\\ \hline \end{tabular} \] Here, \(R-H\) is the alkane, \(Init\) is the initiator and the dot (.) represents a free radical. The reactant \(ROO.\) is a peroxy radical, which can react further, leading to the oxidation of additional alkane molecules.Impact and Results of Catalytic Oxidation of Alkanes
The catalytic oxidation of alkanes facilitates several outcomes that can be quite impactful: - It increases the efficiency of oxidation reactions by reducing the energy input (temperature) required for these reactions, which makes them more accessible and practical for industrial applications. - It also leads to a broader diversity of products. Depending on the type of catalyst used, different intermediates and final products can be formed. Hence, the catalytic process allows us to manipulate the reaction to obtain the desired compounds. However, it's significant to mention few challenges associated with this process: - The catalysts employed can suffer from deactivation over time due to sintering, leaching, and other causes. This issue needs to be consistently addressed in the industrial setting. - Certain conditions, like the presence of water, can hinder the catalytic process. Therefore, the reaction conditions must be carefully optimised to ensure the catalyst's best performance.Uses and Importance of Catalytic Oxidation in Alkanes
The catalytic oxidation of alkanes has found a huge array of applications:- Arising Alternative Fuels: This process serves a crucial role in converting methane and other light alkanes into liquid fuels and more valuable chemicals, thereby utilising abundant natural gas reserves effectively.
- Reduction of Pollutants: It plays a considerable part in the removal of volatile organic compounds (VOCs) and other pollutants from the environment by oxidising them into less harmful substances like \(CO_2\) and \(H_2O\).
- Chemical Synthesis: Catalytic oxidation opens pathways to a broad range of functionalised organic compounds, greatly expanding possibilities of synthetic chemistry.
Insights into Controlled Oxidation of Alkanes
Diving deeper into the subject of alkane oxidation, let's introduce the concept of controlled oxidation, a process designed to direct the oxidation of alkanes towards useful intermediates without fully converting them to carbon dioxide and water. This approach aims to maximise the value obtained from the oxidation reaction and opens the door to potential applications in organic synthesis.Steps Involved in the Controlled Oxidation of Alkanes
Controlled oxidation entails carrying out the oxidation in a manner that allows the isolation of particular oxidation products. The core steps can be summarised as follows:Step 1: Selection of alkane and oxidising agent. Step 2: Deciding on a suitable catalyst to promote the reaction. Step 3: Running the reaction under controlled conditions (temperature, pressure, time). Step 4: Halting the reaction at a stage before full oxidation to CO2 and H2O. Step 5: Isolating and purifying the product.Careful preservation of conditions, such as temperature and pressure, can ensure the production of intermediate compounds instead of complete combustion products. A standard example of controlled oxidation can be observed in the conversion of methane to methanol: \[ CH_4 + \frac{1}{2}O_2 \rightarrow CH_3OH \] This reaction, catalysed by transition metals like copper (II), is one of the most studied in the field of alkane oxidation due to methane's abundance in natural gas.
Significance of Controlled Oxidation in Organic Chemistry
Controlled oxidation processes are of substantial interest in the field of organic chemistry, where selective transformation of simple hydrocarbons into more complex, functionalised molecules is a critical endeavour.
Analysing the Stability and Reactions in Controlled Oxidation
When investigating the oxidation of alkanes, it's necessary to understand the strength of the carbon-hydrogen (C-H) bond in the alkane, which measures the thermal stability. The C-H bond in alkanes is among the strongest single bonds in organic chemistry, averaging a bond energy of around 418 kJ mol\(^{-1}\). This strong bond, combined with the absence of functional groups in alkanes, accounts for their relative chemical inertness and resistance to oxidation. The challenge in controlled oxidation lies in selectively breaking the C-H bonds and manipulating the intermediates formed to achieve desired outcomes. Different reaction routes can be followed, leading to the formation of different products. A primary determinant of the reaction pathway is the nature of the oxidising agent and catalyst used, dictating whether the reaction will be a radical mechanism or a concerted one. For instance, in a free-radical mechanism: \[ R-H + O \longrightarrow R. + HO. \] \[ R. + R. + O_2 \longrightarrow RR + O_2 \] Here, \(R.\) is an alkyl radical, a highly reactive species produced when hydrogen is abstracted from the alkane. A concerted mechanism, on the other hand, involving an oxygen-insertion step, usually leads to alcohol (\(R-OH\)) or ketone (\(R-CO-R\)) products. Understanding these mechanisms and the factors that influence them is crucial for achieving control over alkane oxidation processes and expanding their utility in organic chemistry.Investigating the Oxidation of Alkanes Mechanism
An area that piques significant interest in the study of alkanes is their resistance to chemical reactions due to their inert nature, particularly oxidation reactions. To unlock the mystery of alkanes' reactivity, we delve into the oxidation mechanism of alkanes.Step by Step Analysis of Oxidation of Alkanes Mechanism
Despite the inertness of alkanes, under certain conditions they will react with oxygen. This oxidation, which is essentially a combustion reaction, forms the basis for most of our world's energy production. Below are the standard steps involved in the oxidation of alkanes function:Step 1: Initiation – The reaction begins with the rupture of the O=O bond in molecular oxygen to produce two separate oxygen atoms. Step 2: Propagation – One of these oxygen atoms reacts with an alkane molecule to form an alkyl radical and a molecule of water. Step 3: Further Propagation – The alkyl radical can then react with a second oxygen molecule to produce an alkylperoxy radical. Step 4: Termination – Various termination routes can ensue involving the alkyl and alkylperoxy radicals, leading to a range of combustion products.A simplified reaction is outlined below: \[ R-H + O. \rightarrow R. + OH. \] In this equation, \(R-H\) stands for the alkane and \(O.\) signifies an oxygen atom.
Factors Influencing the Oxidation of Alkanes Mechanism
The oxidation mechanism of alkanes is influenced by several key factors: - Temperature - The rate of oxidation increases with temperature due to a corresponding increase in the kinetic energy of the molecules. - Presence of a Catalyst - The presence of a catalyst can significantly boost the reaction rate by providing alternative reaction pathways with lower activation energies. For example, transition metal complexes are often used as catalysts for the oxidation of alkanes. - Pressure - Higher pressure conditions favour the oxidation of alkanes by increasing the frequency of collisions between reactant molecules. - Oxygen Concentration - An abundance of oxygen contributes to a higher reaction rate as more oxygen molecules will be available to react with the alkane, consequentially forming more reactive intermediates.Practical Examples of Oxidation of Alkanes Mechanism
Arguably, the most prevalent practical example of alkane oxidation is the burning of hydrocarbon fuels. In this context, the oxidation of alkanes serves a vital role in energy production. Take methane, the primary component of natural gas, for instance: \[ CH_4 + 2O_2 \rightarrow CO_2 + 2H_2O \] When methane combusts in the presence of an open flame (a source of high energy), it reacts rapidly with atmospheric oxygen to produce carbon dioxide and water, releasing considerable amounts of energy in the process. In another example, propane, commonly used for home heating and cooking, is oxidised in an analogous manner: \[ C_3H_8 + 5O_2 \rightarrow 3CO_2 + 4H_2O \] Once again, the combustion of propane liberates a large quantity of energy, along with carbon dioxide and water. These examples demonstrate the fundamental importance of the oxidation of alkanes mechanism in producing the energy that powers our lives every day. Nonetheless, the challenge lies in making these reactions clean and sustainable to reduce their environmental impact.Conversion: Oxidation of Alkanes to Alcohol
An intriguing transformation process is the application of controlled oxidation of alkanes to obtain alcohols. This process converts simple hydrocarbons into more useful molecules by introducing an -OH group, which drastically changes the chemical properties of the resulting compound and opens up avenues for further chemical modifications.Steps Involved in Oxidation of Alkanes to Alcohol
An operation like the oxidation of alkanes to alcohol is a carefully calibrated process that allows chemists to convert readily available and abundant feedstocks (like methane in natural gas) into much more valuable and versatile chemicals. To carry out this process effectively, the following steps are taken:Step 1: Choosing an effective oxidising agent. A common choice is molecular oxygen (O2). Step 2: Employing a suitable catalyst to mediate the process. Transition metals like copper or silver are frequently used. Step 3: Implementing the oxidation reaction under carefully controlled conditions (e.g., maintaining a precise temperature and pressure). Step 4: Isolating the desired product – in this case, the alcohol – from the reaction mixture.One might represent the general reaction as follows: \[ R-H + \frac{1}{2}O_2 \rightarrow R-OH \] Here, \(R-H\) signifies the alkane being oxidised, and \(R-OH\) denotes the resulting alcohol.
Impact of Conversion Process: Oxidation of Alkanes to Alcohol
Implementing the transformation of alkanes to alcohols has far-reaching implications, not just for chemistry, but for the broader society and environment. These impacts are:Sustainability: Transforming natural gas (dominated by methane) - an underutilised resource into value-added products such as alcohols reduces reliance on oil for chemical feedstocks and promotes the utilisation of sustainable resources.
Economic Impact: The produced alcohol can be used to generate a myriad of valuable chemicals which can be converted into polymers, pharmaceutical ingredients, and many more. This boosts the economic value of the raw material.
Environmental Impact: While oxidation reactions typically involve the release of a certain amount of CO2, the controlled oxidation of alkanes to alcohol can be more environmentally friendly, especially if precautions are taken to capture and recycle the generated CO2.
Real-World Applications of Converting Alkanes to Alcohol through Oxidation
There are numerous applications for alcohols generated from the controlled oxidation of alkanes. These include, but are not limited to, their use:- As solvents in a range of industrial and laboratory processes.
- In the synthesis of other chemicals. Alcohols are versatile synthetic intermediates that can be converted to aldehydes, ketones, acids, esters, and ethers, amongst others.
- As fuels. Certain alcohols like methanol and ethanol are used as clean-burning fuels in some applications.
- In making consumer goods. Alcohols are common ingredients in cosmetics, cleaning products, inks, and paints.
Oxidation of Alkanes - Key takeaways
- Oxidation of Alkanes Meaning: Oxidation of alkanes refers to a chemical reaction where alkanes are combined with oxygen to form complex partial-oxidation products such as alcohols, ketones, and acids. These products serve crucial roles in various chemical and biological processes.
- Oxidation of Alkanes Applications: The oxidation of alkanes is a fundamental reaction driving many industrial processes, including energy production, pollution control, and the making of polymers. Examples of applications include air heating for buildings, industrial heat production, use in internal combustion engines, and as intermediate products in various syntheses.
- Catalytic Oxidation of Alkanes: This refers to a technique that uses a catalyst to speed up the oxidation process of alkanes. Catalytic oxidation increases the efficiency of oxidation reactions and leads to a broader diversity of products. However, the catalysts used can degrade over time and certain conditions can hinder the process.
- Controlled Oxidation of Alkanes: This is a process where the oxidation of alkanes is directed towards useful intermediates without fully converting them to carbon dioxide and water. This strategy maximizes the value obtained from the oxidation reaction and is of significant interest in the field of organic chemistry.
- Oxidation of Alkanes Mechanism: Understanding the mechanism of alkane oxidation involves studying the strength of carbon-hydrogen (C-H) bonds in the alkane, which measures the thermal stability and influences the reaction pathways. The challenge in controlled oxidation lies in selectively breaking the C-H bonds and manipulating the intermediates formed to achieve desired outcomes.
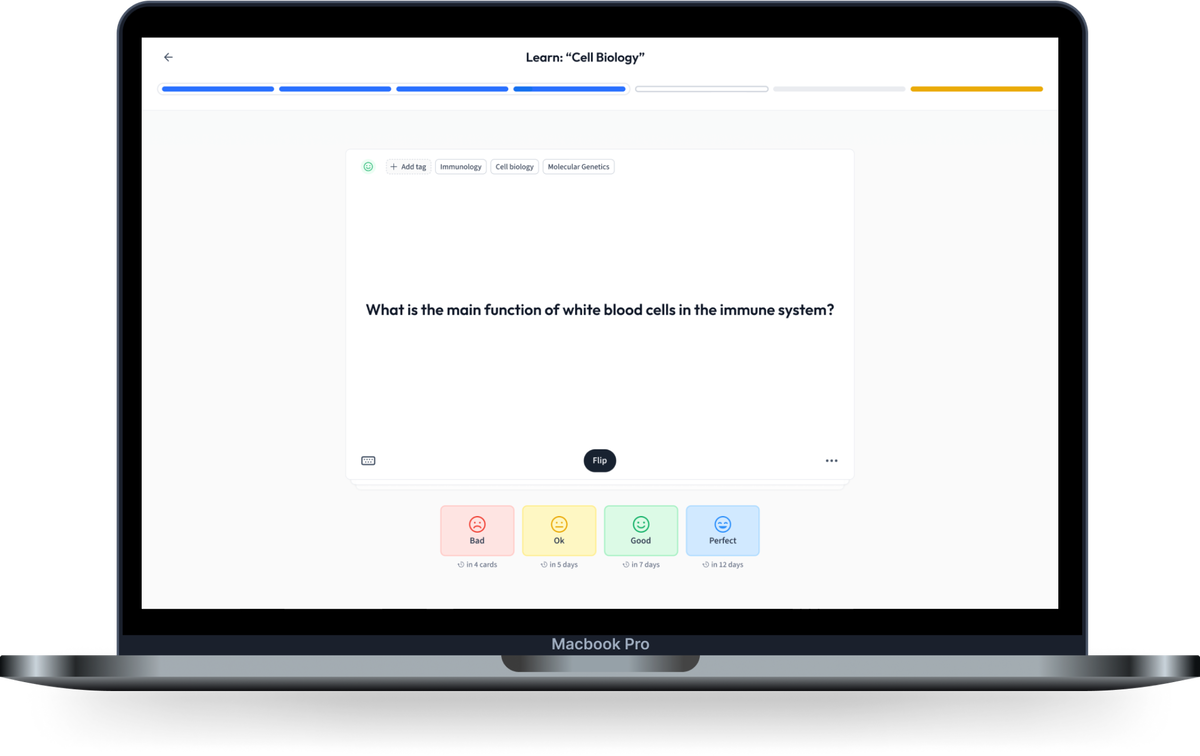
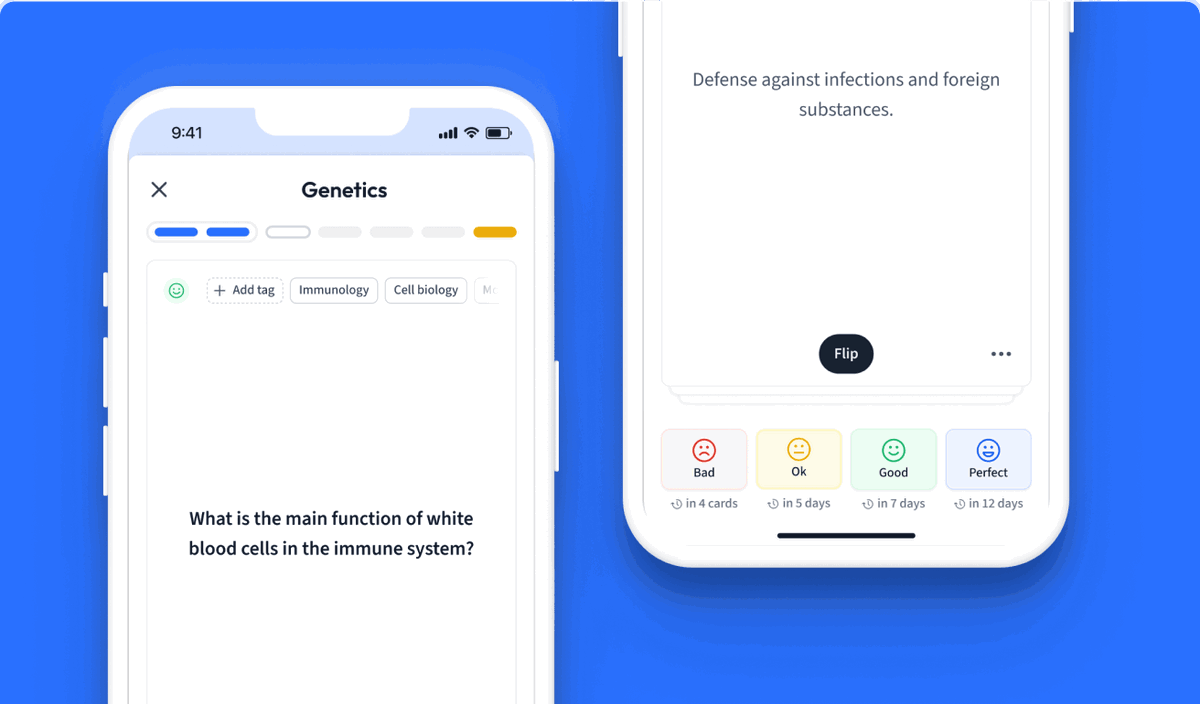
Learn with 14 Oxidation of Alkanes flashcards in the free StudySmarter app
We have 14,000 flashcards about Dynamic Landscapes.
Already have an account? Log in
Frequently Asked Questions about Oxidation of Alkanes
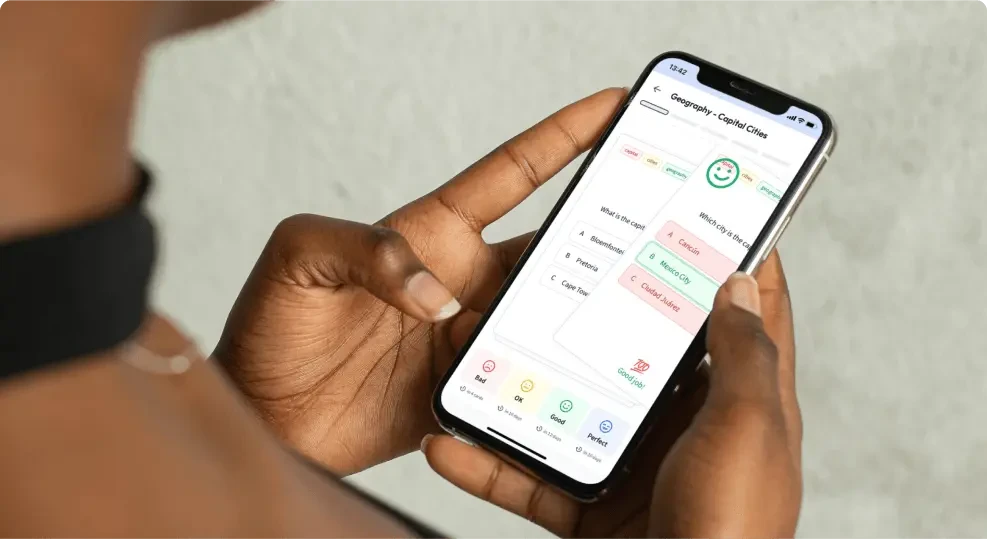
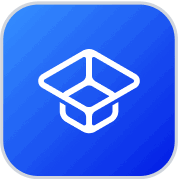
About StudySmarter
StudySmarter is a globally recognized educational technology company, offering a holistic learning platform designed for students of all ages and educational levels. Our platform provides learning support for a wide range of subjects, including STEM, Social Sciences, and Languages and also helps students to successfully master various tests and exams worldwide, such as GCSE, A Level, SAT, ACT, Abitur, and more. We offer an extensive library of learning materials, including interactive flashcards, comprehensive textbook solutions, and detailed explanations. The cutting-edge technology and tools we provide help students create their own learning materials. StudySmarter’s content is not only expert-verified but also regularly updated to ensure accuracy and relevance.
Learn more