Understanding Ether Reactions in Organic Chemistry
Delve into the fascinating world of ether reactions, an essential part of your study of organic chemistry. Ethers play a vital role in numerous chemical reactions and are widely used in both laboratories and industry. Gaining a thorough understanding of ether reactions is crucial if you’re pursuing a path in chemistry.
Introductory Guide: Alcohol to Ether Reaction
In organic chemistry, ethers are commonly synthesised through the reaction of alcohols. This is known as the alcohol to ether reaction, or more commonly, dehydration of alcohols. This reaction is a versatile process, easily tailored and controlled to produce a wide range of different ether compounds.
An ether is a type of organic compound where an oxygen atom is attached to two alkyl or aryl groups.
Essential Steps in Alcohol to Ether Reaction
The process, the alcohol to ether reaction, has several key steps. Understanding these will help you grasp how these reactions happen at a molecular level and will aid in designing or predicting organic reactions.
- Firstly, two alcohol molecules react with each other in the presence of an acid catalyst (commonly sulfuric acid), to form water and an alkene.
- Secondly, the newly formed alkene reacts with another alcohol molecule again in the presence of an acid catalyst to form a different type of ether.
Real-world Cases: Alcohol to Ether Reaction Examples
An example of an ether reaction in real-world organic chemistry is the synthesis of diethyl ether (or simply ether) from ethanol. In this reaction, two ethanol molecules react with each other in the presence of sulfuric acid to form diethyl ether and water.
Exploring the Ether Formation Reaction
Apart from the alcohol to ether reaction, there's another frequently used method in ether's formation. This is the "Williamson Ether Synthesis." Named after Alexander William Williamson, this method serves as a powerful and versatile tool within organic chemistry.
The Williamson Ether Synthesis reaction involves the reaction of a metal alkoxide \( R-O^-M^+ \) with an alkyl halide \(R'-X\) to produce an ether \( R-O-R'\).
Detailed Process of Ether Formation Reaction
The process of ether formation via the Williamson Ether Synthesis involves two major steps.
- First, the metal alkoxide ion is produced by reacting an alcohol with a strong base. The alcohol loses a proton, and a negative charge is located on the oxygen atom.
- Second, this newly formed ion will attack an alkyl halide molecule, ultimately forming an ether after losing a halide ion.
Examples of Ether Formation Reaction in Chemistry
A simplistic example of the Williamson Ether Synthesis is the reaction of ethoxide ion with methyl iodide to form methoxyethane.
Notably, this reaction can be used to prepare unsymmetrical ethers, which the dehydration of alcohol process may struggle to achieve.
From Ether to Alcohol: Demystifying the Reversal Reaction
You've already explored how ethers are synthesised from alcohols; now, it's time to reverse that process and investigate how alcohols can be obtained from ethers. This shift from ethers to alcohols is crucial in organic chemistry and extensively applied in laboratory and industrial settings. By dissecting this reaction, you'll gain a deeper understanding of how organic molecules can be manipulated through chemical transformations.
Basics of the Ether to Alcohol Reaction
Converting ethers back to alcohols is a common situation in the world of organic chemistry. You will often find such reversals in many chemical pathways when specific molecular structures have to be achieved. This reaction is typically accomplished through the application of an acid. This process is called the Acid-Catalysed Hydrolysis of ether.
The Acid-Catalysed Hydrolysis of ether is a reaction where an ether reacts with a water molecule in the presence of an acid to form alcohol and a corresponding acid.
This process is a simplified way to convert relatively reactive ether molecules into more stable and less reactive alcohol compounds. To summarize:
- An ether molecule (R-O-R') is reacted with water under acidic condition.
- The bond between the oxygen and one of the alkyl or aryl groups in ether is cleaved, resulting in an alcohol molecule and a positive organic ion.
- The positive organic ion then combines with a negative ion from the acid to give the corresponding alcohol.
Ether to Alcohol Reaction Mechanism
The reaction mechanism provides detailed information about how chemical bonds form and break during the course of a reaction by going through a series of intermediate steps.
The ether to alcohol reaction mechanism is a two-step process:
- Step 1: Protonation of Ether - Here, the proton from the acid catalyst protonates the oxygen atom of the ether. This results in the formation of a positive ion.
- Step 2: Nucleophilic Attack - In this step, a water molecule attacks one of the alkyl groups bonded to the oxygen atom, forming an intermediate ion. The bond between the oxygen atom and the alkyl group is cleaved, resulting in an alcohol.
Practical Ether to Alcohol Reaction Examples
In order to cement your understanding, let's walk through some real-world examples with simple and familiar molecules.Take for instance diethyl ether and water in the presence of acid. The diethyl ether reacts with water under the acidic condition to yield ethanol and hydronium ions. The direction of this reaction is driven towards the formation of ethanol, a stable alcohol.
Another example is the reaction of dimethyl ether with water in the presence of acid. This results in the formation of methanol and hydronium ions. Again, the product methanol is much more stable than the initial ether compound.
Grignard Reaction with Ether: A Detailed Study
When it comes to understanding ethers and their unique chemistry, one vital reaction to comprehend is their interaction with Grignard reagents, a cornerstone of organometallic chemistry. These reactions hold significant importance in developing complex organic structures in both academic research and industrial applications.
Grignard Reaction with Ether: Understanding its Significance
Named after Victor Grignard, a French chemist who discovered it, the Grignard reaction is a powerful tool in organic chemistry. In this reaction, a specially prepared magnesium-containing compound, known as a Grignard reagent, reacts with multiple classes of organic compounds, including ethers, to form new compounds.
A Grignard reagent is an organomagnesium compound typically represented as \(RMgX\) where \(R\) is an alkyl group and \(X\) is a halogen atom.
Grignard reactions with ether are particularly significant since ethers often serve as the solvent for these reactions. It’s also important to note that Grignard reagents can react with ethers under certain conditions, breaking open the ether in an oxidative insertion process.
Process of a Grignard Reaction with an Ether
Grignard reactions occur under basic conditions, often with ether being employed as a solvent to help stabilise the highly reactive Grignard reagent. When it comes to the reaction process:
- Initially, the Grignard reagent is prepared by reacting an alkyl or aryl halide with magnesium metal, typically in an ether solvent.
- Once the Grignard reagent is formed, it's then reacted with an ether. The magnesium atom in the Grignard reagent acts as a strong nucleophile, attacking the electrophilic carbon atom in the ether.
- This results in an oxidative insertion of the Grignard reagent into the C-O bond of the ether, followed by a reductive elimination step which removes the magnesium halide. The end product is a new compound with a larger alkyl or aryl group.
Materialising Concepts: Grignard Reaction with Ether Examples
It's often easier to understand these kinds of reactions with concrete examples.
Consider butylmagnesium chloride, a typical Grignard reagent, reacting with diethyl ether. In this reaction, the butyl group from the Grignard reagent attacks one of the ethyl groups in diethyl ether. The product is butylethyl ether and ethylmagnesium chloride.
In laboratory settings, dimethyl ether (DME) can react with a Grignard reagent to produce a new ether in which one of the methyl groups is replaced by a new alkyl group from the Grignard reagent.
For example, if you react dimethyl ether with phenylmagnesium bromide, it will yield anisole and methylmagnesium bromide. Anisole has one phenyl and one methyl group attached to the oxygen atom, verifying the outcome of the substitution process.
In essence, Grignard reactions with ethers allow for the versatile modification and enhancement of organic compounds, a tool of immense value to synthetic chemists.
Williamson Ether Reaction and its Impact in Organic Chemistry
The Williamson Ether synthesis holds an esteemed position within the realm of organic chemistry. You may ask why? Primarily, because it is an efficient and straightforward method of creating ethers, which play a pivotal role in industries and scientific research. This reaction’s discovery was a landmark for organic synthesis and it still remains invaluable to organic chemists across the globe.
Discovering the Williamson Ether Reaction
Named after British chemist Alexander William Williamson who first described it in 1850, the Williamson Ether Synthesis is a fundamental reaction in organic chemistry for the formation of ether.
A Williamson Ether Synthesis reaction involves the attack of a nucleophilic alkoxide ion on a primary haloalkane or tosylate under basic conditions to give a symmetrical or unsymmetrical ether, along with a small amount of halide or p-toluene sulfonate as by-product.
The popularity of this reaction amongst chemists comes from its high yield and ability to produce complex ethers with precise control over their structure. The main strengths of the Williamson Ether Synthesis are its:
- Reactions which occur under mild conditions.
- Flexibility and broad scope.
- Use of readily available starting materials.
- Cheap and convenient operational steps.
The Reaction Mechanism of Williamson Ether Synthesis
Now, let’s dive deeper into the heart of the Williamson Ether Synthesis reaction mechanism. To fully understand it, we need to examine the entire process step by step. This reaction can be divided into two stages: preparation of the alkoxide and the nucleophilic substitution.
- Step 1: Preparation of alkoxide - This reaction begins with a metal alkoxide being formed through the reaction between an alcohol and an alkali metal, such as sodium or potassium. The alkoxide ion that is produced is a potent nucleophile.
- Step 2: Nucleophilic substitution – The nucleophilic alkoxide ion attacks the primary haloalkane or tosylate, expelling the halide or tosylate ion and forming the ether product.
Scenario-based Learning: Williamson Ether Reaction Examples
Let’s illustrate this mechanism with specific examples. Understanding real-world scenarios provides better clarity for abstract concepts.
Consider a reaction between ethanol and bromoethane under the basic conditions of sodium ethoxide. Working as a strong nucleophile, sodium ethoxide reacts with bromoethane to generate diethyl ether and sodium bromide. The alcohol produced in this reaction is diethyl ether, which finds extensive use in industries as a solvent and anesthetic.
If you have butan-1-ol reacting with 2-bromopropane in the presence of a base like sodium hydroxide, you can produce an unsymmetrical ether. The end product, 2-methoxypropane, is an important industrial chemical which has various applications, such as an octane booster in gasoline.
In essence, this highly adaptable reaction is a cornerstone to build complex structures in the world of chemistry and cannot be underestimated!
Examining the Ether Synthesis Reaction
As you delve deeper into the fascinating world of chemistry, the synthesis of ethers is one valuable chapter you must master. Ethers, characterised by an oxygen atom connected to two alkyl or aryl groups, are notable for their widespread application in medicines, solvents, and even as fuel additives.
The Meaning and Role of the Ether Synthesis Reaction
Simply defined, an ether synthesis reaction is a process where ethers are produced from simpler organic compounds. Two main methods are employed for ether synthesis: the Dehydration of alcohols and the Williamson Ether Synthesis. Notably, the former is suitable for producing symmetrical ethers, while the latter works well for both symmetrical and unsymmetrical ethers.
Dehydration of alcohols is an elimination reaction where an alcohol loses a molecule of water to form an ether. The Williamson Ether Synthesis, on the other hand, involves the nucleophilic substitution reaction between a haloalkane or halogenoarene and an alcohol in the presence of a base.
These synthesis reactions are crucial as they pave the way for the creation of a myriad of complex organic compounds, which are integral to various industries including pharmaceuticals, agriculture and plastics. The ability to control and direct these ether synthesis reactions opens up a diverse range of possibilities for chemists, allowing them to build specific compounds with selected functional groups.
How does the Ether Synthesis Reaction Work?
To understand the overall ether synthesis reaction, let’s delve into the mechanism of each method. Dehydration of an alcohol to form an ether is a step by step process that unfolds in the following way:
- Protonation of the alcohol to form a good leaving group.
- Nucleophilic attack of another alcohol molecule on the protonated alcohol, leading to the formation of the intermediate.
- Departure of the water molecule and formation of the ether.
Meanwhile, the Williamson ether synthesis follows its own distinct pathway:
- Deprotonation of the alcohol to form the alkoxide anion.
- Nucleophilic attack of the alkoxide on the haloalkane or tosylate.
- Displacement of the halide or tosylate ion to form the ether.
Practical Examining of Ether Synthesis Reaction Examples
To enhance your understanding of these reactions, let's explore some practical examples.
For instance, in the dehydration of ethanol with sulphuric acid, a diethyl ether is produced. The reaction is typically carried out at around 140°C.
In contrast, the Williamson Ether Synthesis might involve the reaction of sodium methoxide (formed from methanol and sodium) with chloroethane to form ethyl methyl ether.
From Methanol to Dimethyl Ether: An Insight into the Reaction Process
Another impressive transformation that really showcases the capabilities of ether synthesis reactions is the conversion of methanol to dimethyl ether. This is an essential reaction in the production of clean biofuels and presents a viable pathway for the valorisation of surplus, renewable methanol.
Understanding the Methanol to Dimethyl Ether Reaction
The synthesis of dimethyl ether from methanol is typically carried out in the presence of a catalyst to lower the required temperature and pressure. This reaction is an example of alcohol dehydration, which results in the loss of a water molecule from two methanol molecules to form dimethyl ether.
The entire reaction process could be summarised in the three crucial steps:
- Protonation of the methanol molecules by the catalyst.
- Nucleophilic attack of one methanol molecule on the protonated methanol, leading to the formation of the ether and water.
- Removal of water from the active sites, allowing the catalyst to participate in a new reaction cycle.
Methanol to Dimethyl Ether Reaction Conditions for Success
To ensure that dimethyl ether is produced efficiently from methanol, certain conditions must be met. The operating conditions, particularly temperature, pressure, and the presence of a suitable catalyst, are crucial for the success of this reaction.
- Temperature: This reaction is usually carried out at elevated temperatures, ranging from 200 to 300 degrees Celsius.
- Pressure: A moderate pressure, around 8 to 20 bars, is preferred to keep the reactants in the liquid phase during the reaction.
- Catalyst: Typically, solid acid catalysts are used since they facilitate the protonation of methanol, a key step in the dehydration process.
An understanding of these conditions is central to controlling the reaction, allowing you to optimise the yield and purity of the dimethyl ether product.
Understanding Reaction Conditions for Ethers
In the realm of organic chemistry, preparing ethers often involves processes such as dehydration of alcohols or Williamson ether synthesis. Optimising these reactions to effectively produce the required ether compound requires a comprehensive understanding of the various reaction conditions and their influence on the reaction mechanism.
Deep Dive: Ether Reaction Conditions
In organic chemistry, reactions rarely occur in isolation. They are influenced by a variety of factors known as reaction conditions. These include temperature, concentration of reagents, presence of catalysts, reaction duration, and more. Ether reactions such as the dehydration of alcohols or the Williamson ether synthesis are not exceptions. To achieve precise control over the formation of the required ether product, each reaction condition must be monitored and meticulously controlled.
Temperature affects the speed of reactions by providing particles with the required energy to reach the activation energy. A high temperature generally increases the speed of reactions as more particles have enough kinetic energy to react. However, in some ether reactions, it may also promote unwanted side reactions, thus a balance must be achieved.
Reagent concentration is another crucial factor, as a high concentration leads to an increased frequency of collisions between reactant particles, thereby accelerating the reaction rate. The concentration of reactants and products should ideally be determined based on the stoichiometry of the reaction.
Catalysts are substances that increase the rate of a reaction by providing an alternative reaction pathway with a lower activation energy. Catalysts are not consumed during the reaction, and they do not alter the final composition of the products.
Reaction duration is often as important as other reaction conditions. In many cases, limiting the reaction time or applying specific timing patterns can lead to higher yields or more selective production of the desired ether product.
The choice and management of these reaction conditions are often a trade-off and require careful consideration and experience. Therefore, understanding these conditions is pivotal to successful and efficacious ether reactions.
Optimum Ether Reaction Conditions: A Comprehensive Guide
There isn’t a one-size-fits-all rule for the selection of optimal conditions for ether reactions. Conditions can vary depending on factors such as the exact reaction taking place, the specific ethers being synthesised, and the restrictions of the experimental setup. However, we can still draw some general principles for condition selection.
For example, when synthesising ethers through the dehydration of alcohols, a typical condition selection might involve the following considerations:
- Temperature: Usually, a temperature around 110-130 degrees Celsius is opted for primary and secondary alcohols while for tertiary alcohols, this can be lowered to around 100 degrees Celsius. Choosing the right temperature is essential to balance the reaction rate and unwanted side reactions.
- Acid concentration: A concentrated strong acid such as sulphuric acid or phosphoric acid is often used to protonate the alcohol and make it a good leaving group.
- Reaction duration: The reaction is allowed to proceed until the required conversion has been reached, which could be determined through an analytical technique such as gas chromatography.
In the case of the Williamson ether synthesis, the following reaction conditions are typically considered:
- Temperature: This reaction is usually performed at room temperature to avoid side reactions.
- Base: A large excess of a strong base such as sodium hydride or potassium hydride is used to ensure full conversion of the alcohol into the alkoxide ion.
- Solvent: A suitable polar aprotic solvent such as dimethyl sulfoxide (DMSO) or tetrahydrofuran (THF) is used to dissolve the reactants and provide a medium for the reaction to occur.
The Role of Reaction Conditions in Successful Ether Reactions
The reaction conditions, as outlined above, play an instrumental role in dictating the success and efficiency of ether formation reactions. By optimising these conditions, chemists can enhance yields, minimise unwanted by-products, and improve the overall reaction efficiency.
Take the influence of temperature as an example. While elevating the temperature can speed up a reaction by providing sufficient energy for the reactants to overcome the activation energy, it may also boost side reactions. Hence, it's a fine balance between achieving the desired reaction rate and minimising undesirable side reactions.
Catalysts can drastically transform a reaction by lowering the activation energy, thereby speeding up the reaction rate tremendously. Chosen wisely, a catalyst can lead to significant improvements in the production of ethers, permitting the reactions to proceed under milder conditions and often yielding purer products.
Lastly, the concentration and type of the reagents profoundly affect the reaction pathway. An excess of one reagent over the other can drive the reaction towards the desired product. Meanwhile, some reagents can serve two purposes – playing the role of a reactant and at the same time facilitating the reaction mechanism.
In conclusion, understanding how each of these conditions impacts ether reactions is foundational knowledge for any chemist. It allows the development and optimisation of novel reactions, paving the way for new ethers with unique properties.
Ether Reactions - Key takeaways
- Ether to alcohol reaction is a two-step process involving protonation of ether and a nucleophilic attack, leading to the formation of alcohol.
- Grignard reaction with ether is significant in the formation of complex organic structures. It involves a nucleophilic attack by the Grignard reagent on the ether, leading to new compound formation.
- Williamson Ether Synthesis, discovered by Alexander William Williamson, is a process of creating ethers through a nucleophilic alkoxide ion attack on a primary haloalkane or tosylate under basic conditions.
- Ether synthesis reactions include the Dehydration of alcohols and the Williamson Ether Synthesis, essential in producing various complex organic compounds.
- The conversation of methanol to dimethyl ether is essential in biofuel production and involves three steps: protonation of the methanol molecules, nucleophilic attack forming the ether and water, and removal of water.
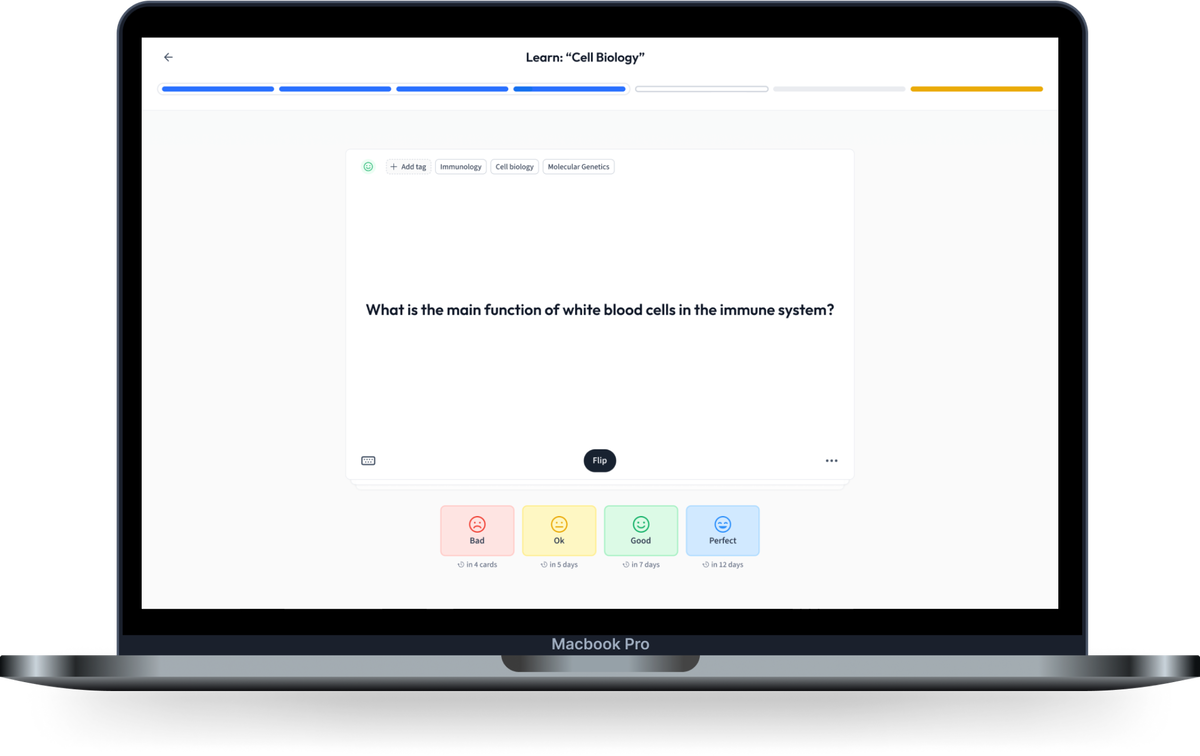
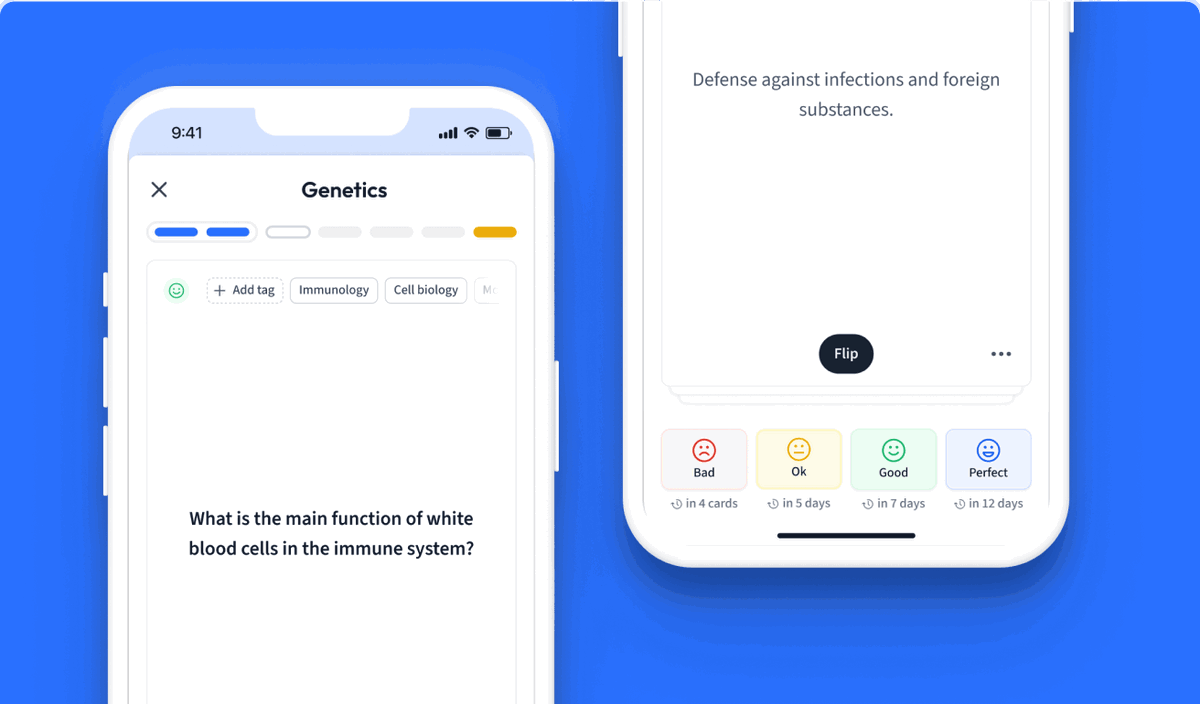
Learn with 51 Ether Reactions flashcards in the free StudySmarter app
We have 14,000 flashcards about Dynamic Landscapes.
Already have an account? Log in
Frequently Asked Questions about Ether Reactions
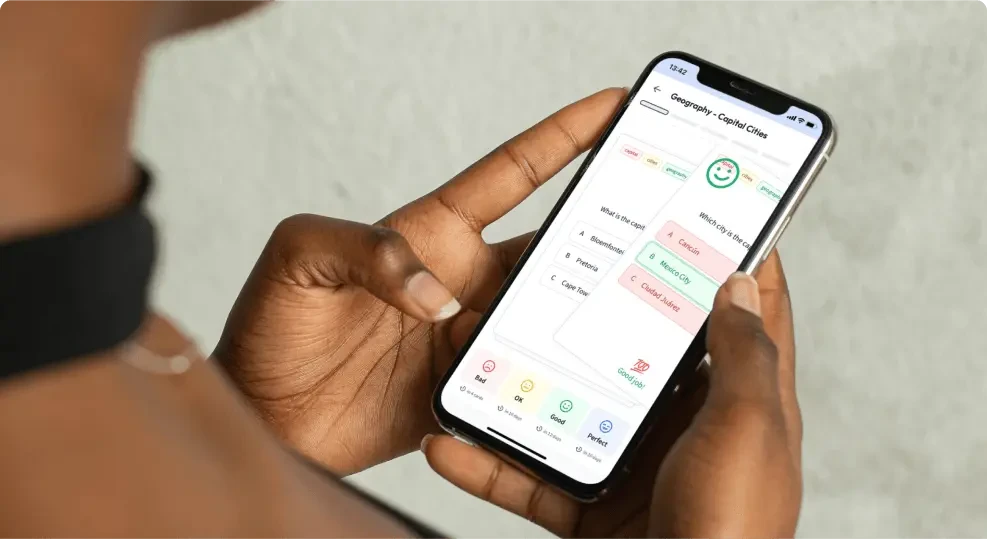
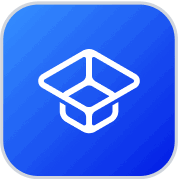
About StudySmarter
StudySmarter is a globally recognized educational technology company, offering a holistic learning platform designed for students of all ages and educational levels. Our platform provides learning support for a wide range of subjects, including STEM, Social Sciences, and Languages and also helps students to successfully master various tests and exams worldwide, such as GCSE, A Level, SAT, ACT, Abitur, and more. We offer an extensive library of learning materials, including interactive flashcards, comprehensive textbook solutions, and detailed explanations. The cutting-edge technology and tools we provide help students create their own learning materials. StudySmarter’s content is not only expert-verified but also regularly updated to ensure accuracy and relevance.
Learn more